Tracking the flow of hippocampal computation: Pattern separation, pattern completion, and attractor dynamics
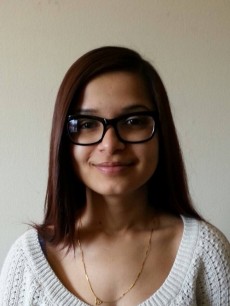
Pooja Acharya
Department of Biology
Lake Forest College
Lake Forest, IL 60045
Ever wondered what your life would be like if you were unable to form new memories? Such was the case of Henry Molaison also known as patient H.M. Patient H.M. who suffered from seizures underwent a bilateral surgery to remove his medial temporal lobe that consisted of most of the hippocampal formation. While this helped with the seizures, it left him unable to form declarative memories.
The case mentioned above, is one of many that illustrates the importance of the hippocampal formation, particularly, the structure known as hippocampus. The hippocampus, named after its resemblance to the seahorse, is an important structure in the vertebrae brain. It’s very important in memory formation and has been implicated in memory storage and retrieval as well. Information from other areas of the brain flow through the hippocampus in a sequence that has been named the tri-synaptic circuit. Figure 1. Shows the overall flow of the inputs and outputs of information in the tri-synaptic circuit.
Beginning at the entorhinal cortex, projections go to the hippocampal formation via the perforant path. Perforant path axons project to the dentate gyrus (DG) granule neurons, then from there, send axons along the mossy fiber pathway to pyramidal neurons in area CA3. The pyramidal cells in area CA3 then project along the Schaffer collaterals to pyramidal neurons in area CA1. The pyramidal cells in area CA1 then project back to the entorhinal cortex. This describes how information can loop through same areas of the hippocampus as inputs and outputs.
Current research is now looking at the exact mechanisms of information flow in the hippocampus. A review article by Knierim and Neunuebel, “Tracking the flow of hippocampal computation: Pattern separation, pattern completion, and attractor dynamics”, published in 2015, sought to understand how information is processed in the hippocampus. Specifically, they were looking to see if their results matched the theories proposed by David Marr in the late 1960s’. This theory proposed that the DG’s main function was pattern separation where overlapping inputs of information are transformed into individual information outputs. On the other hand, in the CA3, the process of pattern completion involves retrieving from memory, a complete pattern of information when presented with incomplete or degraded fragments of the pattern. However, the CA3 also tends to display attractor dynamics, which means a set of stable patterns that “attract” neighboring pattern to move toward the same state. Combined with pattern separation from DG inputs, this means the CA3 outputs show both pattern separation and pattern completion. Lastly, CA1 is thought to have a linear relationship with input and output, displaying neither pattern separation nor completion.
In order to evaluate this theory, Knierim performed a series of double rotation experiments in 2002. In these experiments, rats ran on circular tracks, each one with a different visual and tactile texture. In the same room as the track, a black curtain was placed in the periphery with
six global cues on it. After days of being conditioned to run on the track for food, the rats experienced a 4 day sequence of the cues being arranged in either standard configuration or mismatched configuration. In the mismatched configuration, local track cues were rotated counter-clockwise while the global cues were rotated clockwise. There were four different mismatched configuration with the mismatch between local and global cues being 45º, 90º, 135º, and 180º.
Recordings were made from the medial and lateral entorhinal cortex, the anterior thalamus, DG, CA3 and CA1. Results from the recordings revealed that David Marr’s theory was pretty accurate describing the characteristics of the hippocampal information about the inputs and outputs. The first two findings were about the medial and entorhinal cortex and its inputs to the DG. These findings are highly implicit for spatial memory research, as the medial entorhinal cortex input representations are dependent global cues while lateral entorhinal cortex input representations are dependent on local cues. It was also found that the DG does in fact perform pattern separation on its entorhinal cortex inputs. This is found by looking at the changes in inputs from the medial and lateral entorhinal cortex to the DG and how the difference between both areas increased as cue mismatched increased. Consistent with David Marr’s theory, recording the input and output changes in CA3 revealed that it does perform pattern completion as well as pattern separation. Lastly, CA1 was found to represent a combination of entorhinal cortex and CA3 inputs.
In conclusion, using modern research methods, an older theory about hippocampal function was evaluated and was found to be similar to the results of the study. However, the study does not come without caveats, mainly among them is that hippocampal anatomy is far more complex than it described in the article. While the medial and entorhinal cortex are the main inputs to the DG, there are other inputs that were not measured from such as the septum, brain stem, and peririhnal cortex. Another interesting caveat is the role of adult neurogenesis in the DG as the age of the granule cells were not taken into account and it is unknown whether recordings would be affected by immature vs. mature cells. Another major caveat is that the results from the memory task cannot be generalized to all forms of memory. It is only speculation as to how the hippocampal circuit would function if a different behavioral task targeting a different form of memory was employed.
Note: Eukaryon is published by undergraduates at Lake Forest College, who are solely responsible for its content. The views expressed in Eukaryon do not necessarily reflect those of the College.
References
Baumgart, D. C., & Carding, S. R. (2007). Inflammatory bowel disease: Cause and immunobiology. Lancet, 369(9573), 1627-1640.
Bouma, G., & Strober, W. (2003). The immunological and genetic basis of inflammatory bowel disease. Nature Reviews.Immunology, 3(7), 521-533.
Brenner, O., Levanon, D., Negreanu, V., Golubkov, O., Fainaru, O., Woolf, E., et al. (2004). Loss of Runx3 function in leukocytes is associated with spontaneously developed colitis and gastric mucosal hyperplasia. Proceedings of the National Academy of Sciences of the United States of America, 101(45), 16016-16021.
Cadwell, K., Liu, J. Y., Brown, S. L., Miyoshi, H., Loh, J., Lennerz, J. K., et al. (2008). A key role for autophagy and the autophagy gene Atg16l1 in mouse and human intestinal paneth cells. Nature, 456(7219), 259-263.
8