The Role of Horizontal Gene Transfer in Antibiotic Resistance
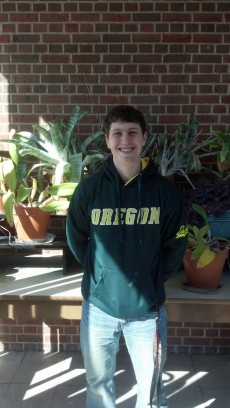
The discovery of the first antibiotic, penicillin, in the early 20th century was a landmark medical breakthrough that helped protect humans and their domesticated animals from bacterial agents. Many believed that this discovery would lead to the elimination of all illnesses and a society of essentially perfect health. However, none of these predictions were true and slowly the “miracle medicine penicillin” became less effective. With the discovery of DNA being the genetic code, scientists determined that some bacteria were resistant to particular antibiotics because of genes that rendered bacteria unaffected by the effects of some antibiotics. Populations contain genetic variation that allows the survival of some individuals of the population when faced with changing environmental factors. Genetic variation is the result of mutations in coding regions of DNA. The introduction of antibiotics into bacterial colonies increased the biological fitness and the frequency of antibiotic resistant genes in bacterial populations. Horizontal gene transfer allows new variants to arise without a mutation in that variant. In addition to antibiotic resistance increasing from natural selection, bacteria can receive genetic material through the process of horizontal gene transfer. The genetic material is received in two forms: a DNA plasmid or a transposon. A transposon is genetic material from one organism that becomes incorporated into the DNA of another organism, while plasmids do not become incorporated into the DNA of the host organism. There are two main mechanisms in which bacteria receive extracellular genes. Transformation is the uptake of genetic material from a dead organism, while conjugation is the transfer of genetic material between two living organisms (Kaiser, 2012). Horizontal gene transfer contributes to the spread of antibiotic resistance through the exchange of genetic material across genera, which increases the potential for a harmful, antibiotic resistant bacteria to develop.
The unnecessary use of antibiotics by humans acts as a selective pressure to increase the frequency of antibiotic resistance genes. When an illness is caused by a microbial, antibiotics are initially effective because the resistance to that antibiotic is either not present or has a low frequency in the population. Although the individual will most likely be healthy after their treatment, the microbes remaining have a higher likelihood of containing the resistance gene. The continuous use of an antibiotic will make it less effective over time because it will increase the frequency of a resistance gene. Hospitals often rotate the use of different antibiotics to try and prevent the frequency of one antibiotic resistance gene from becoming too prevalent or standardized in a bacterial colony. Rotating antibiotics removes the selective pressure favoring one specific type of antibiotic resistance causing the frequency of the resistance gene to decrease. However, this leads to the selection for resistance to another type of antibiotic. One hospital continued to rotate the antibiotics gentamicin and tobramycin. When one became ineffective, the other would be used so resistance to the other decreased. Therefore, fewer patients would contract bacterial infections because an antibiotic with high bacterial resistance was not being used. However, this eventually led to the resistance to both tobramycin and gentamicin (Gerding, 1991). This could have occurred by bacteria receiving each gene that provided resistance against the bacteria, or one gene that is resistant to multiple antibiotics evolved (Salyers & Shoemaker, 2006).
The human colon is home to billions of bacteria (Salyers, Moon, & Schlessinger, 2007). Bacteria rapidly divide, making it likely that many mutations arise from errors in DNA replication. Therefore, the genetic variation in the population increases, creating an “insurance effect,” where a change in the environment may harm a part of the population, but ensures the survival of the population as well (Tyerman, Ponciano, Joyce, Forney, & Harmon, 2013). Since bacteria are ubiquitous in the colon, conjugation is constantly occurring. This conclusion has been supported by bacteria in different genera containing homologous DNA plasmids. Therefore, horizontal gene transfer can occur between species as well as within a population. This can become problematic if harmful bacteria that have been artificially selected for antibiotic resistance happen to be in the colon, where bacteria can transfer the resistance gene to other species of bacteria. Typically, this is not a problem because most bacteria are not harmful, unless bacteria that are a public health concern happen to receive a resistance gene. Individuals that have previously taken antibiotics are less responsive to treatment because their bodies contain more antibiotic resistant bacteria (Gerding, 1991). These bacteria received these genes from disease causing microbes that transferred a resistance gene through conjugation or transformation. The harmless bacteria that are resistant to antibiotics can then pass this gene to harmful bacteria that do not yet have antibiotic resistance. Thus, horizontal gene transfer allows bacteria to indirectly become resistant to antibiotics. However, antibiotics can also accelerate the transfer of antibiotic resistant phenotypes.
The conjugation of an antibiotic resistant gene can be stimulated by the use of antibiotics. The conjugative transposon CTnDOT provides resistance to the antibiotic tetracycline (Jeters, Gui-Rong, Kyung, Shoemaker, & Salyers, 2009; Kyung, Shoemaker, Gardner, & Salyers, 2005). The excision genes and integration genes that allow for CTnDOT to be transferred are stimulated by the use of tetracycline. Thus, the transfer of resistance to tetracycline is accelerated by the presence of antibiotics. CTnDOT is composed of four genes, one is the gene that expresses the tetraycline resistance phenotype (tetQ), and the other three genes regulate the excision of the entire conjugative transposon (Kyung et al., 2005). This entire gene cluster is an operon, which can be influenced by one promoter. In this instance, the operon containing tetQ is promoted by tetracycline (Kyung et al., 2005). Therefore, tetracycline increases the expression of both tetQ and the necessary excision enzymes allowing for tetQ to be transferred. Even if harmful bacteria do not contain CTnDOT, its presence in another bacterium can allow other bacteria to receive it. The over prescribing of antibiotics initially increases frequency of antibiotic resistance through natural selection, but eventually increases the indirect transfer of resistance genes as well. The overuse of antibiotics can prove to be problematic, because its use in agriculture can also harm public welfare.
The use of antibiotics in agriculture can reduce the ability to eliminate bacteria hazardous to human health. Since the mid-1980s, the use of antibiotics on poultry farms has risen by 307% (Sapkota et al., 2011). Antibiotics are utilized to make crops and livestock less susceptible to microbial induced illnesses and cause livestock to grow bigger and faster. Due to the public’s knowledge of the detrimental effects of antibiotic use in agriculture, many prefer to purchase organically farmed products. Sapkota (2011) compared antibiotic resistance frequency on organic and conventional poultry farms. Conventional poultry farms contain greater levels of antibiotic resistant microbes than organic farms because of the lack of antibiotics used at organic farms. In the conventional poultry houses, 81% of E. faecium were resistant, while 12% contained the tetramycin resistance gene in the organic farms. Although 81% of E. faceium were resistant in the non-organic farms, it is surprising that even at the organic farms 12% of the E.faecium bacterial population was tetramycin resistant as well. The organic farms where the study was conducted had previously been converted from conventional poultry farms. Despite the measures taken to be become a certified organic farm, antibiotic resistant bacteria most likely remained and continued to persist. Organic farms do not always use eggs that were hatched on their own farms. Some organic farms receive their eggs from hatcheries that inject eggs with antibiotics to increase the percentage of eggs that hatch (Sapkota et al., 2011). Antibiotic resistance frequencies will continue to persist in organic farms because of external sources of antibiotics. Through mutations and gene transfer, resistance may continue to slowly spread to different species, despite antibiotics not being used on organic farms. The waste produced by livestock can also lead to an increase of antibiotic resistance.
Waste and manure can facilitate the transfer of a variety of genes (Jing, Michel Jr., Sreevatsan, Morrison, & Zhongtang, 2010). Manure is semi-aqueous, which allows microbes to move freely and more frequently exchange genetic material (Jing et al., 2010). Additionally, manure seeps into the ground, allowing bacteria to spread into water supplies and can affect food pyramids (Maal-Bared, Bartlett, Bowie, & Hall, 2013). Although natural selection provides the initial spike in antibiotic frequency, conjugation and transformation allow this genetic material to cross species. Once bacteria seep into the ground, there are countless opportunities for these populations to horizontally transfer genetic material to a variety of microbial species. The most dangerous outcomes involve bacteria that are harmful and end up in water supplies. This could cause a widespread public health concern affecting both humans and the food supplies as well. Since antibiotics also end up in water supplies, it can provide a positive feedback mechanism for the excision and transfer of genetic material that codes for antibiotic resistance, such as CTnDOT (Jing et al., 2010). Hopefully, food companies begin to think about public welfare before profits.
Transformation and conjugation contribute to increasing frequencies of antibiotic resistant genes because of genes transferring between different species. Although natural selection plays a prominent role in increasing the frequency of alleles, transformation and conjugation make the gene available to a wide variety of microbes. The over prescribing of antibiotics in agriculture and human illnesses has largely reduced the effectiveness of antibiotics. If society continues to abuse antibiotics, it will have difficulty treating potentially life- threatening illnesses because many antibiotics will become ineffective. The discovery of antibiotics was one of the greatest medical innovations in human history, while its abuse can lead to the development of one of society’s worst epidemics.
References
Gerding, D. N. (1991). Aminoglycoside resistance and ami- noglycoside usage: Ten years of experience in one hospital. Antimicrobial Agents and Chemotherapy, 35, 1284-1290.
Jeters, R. T., Gui-Rong, W., Kyung, M., Shoemaker, N. B., & Salyers, A. A. (2009). Tetracycline-associated transcriptional regulation of transfer genes of the bacteroides conjugative transposon ctndot. Journal of Bacteriology, 191, 16-16.
Jing, C., Michel Jr., F. C., Sreevatsan, S., Morrison, M., & Zhongtang, Y. (2010). Occurrence and persistence of erythromycin resistance genes (ERM) and tetracy- cline resistance genes (TET) in waste treatment sys- tems on swine farms. Microbial Ecology, 60, 479-486.
Kaiser, G. (2012). Horizontal gene transfer. Retrieved from http://faculty.ccbcmd.edu/courses/bio141/lecguide/ unit1/HGT/HGT.html
Kyung, M., Shoemaker, N. B., Gardner, J. F., & Salyers, A. A. (2005). Regulation of excision genes of the bacte- roides conjugative transposon ctndot. Journal of Bacteriology, 187, 5732-5741.
Maal-Bared, R., Bartlett, K. H., Bowie, W. R., & Hall, E. R. (2013). Phenotypic antibiotic resistance of escherich- ia coli and e. Coli o157 isolated from water, sediment and biofilms in an agricultural watershed in british columbia. Science of the Total Environment, 443, 315-323.
Salyers, A., & Shoemaker, N. B. (2006). Reservoirs of antibiotic resistance genes. Animal Biotechnology, 17, 137-146.
Salyers, A. A., Moon, K., & Schlessinger, D. (2007). The human intestinal tract – a hotbed of resistance gene trans- fer? Clinical Microbiology Newsletter, 29, 25-30.
Sapkota, A. R., Hulet, R. M., Zhang, G., McDermott, P., Kinney, E. L., Schwab, K. J., & Joseph, S. W. (2011). Lower prevalence of antibiotic-resistant enterococci on U.S. conventional poultry farms that transitioned to organic practices. Environmental Health Perspectives, 119, 1622-1628.
Tyerman, J. G., Ponciano, J. M., Joyce, P., Forney, L. J., & Harmon, L. J. (2013). The evolution of antibiotic susceptibility and resistance during the formation of escherichia coli biofilms in the absence of antibiotics. BMC Evolutionary Biology, 13, 1-7.