The Nobel Prize in Physiology or Medicine: A Century of Winners
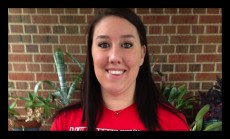
Amber Craghead
Department of Biology
Lake Forest College
Lake Forest, Illinois 60045
Download PDF
*This author wrote the paper as a part of BIOL485: Senior Seminar: The Nobel Prizes under the direction of Dr. Maine.
Over the course of the semester in BIO 485 the Nobel Prizes: A Century of Innovation and Discovery, we have covered the work of several Nobel Laureates who won the Nobel Prize in Physiology or Medicine over the past 100 years. Every week, we carefully examine the original papers written by these laureates and then as a class, we present and discuss the papers in order to have a firm understanding of the importance and implications of the work. Combined, we have read the works of 27 Nobel Laureates of Physiology or Medicine, which is an impressive accomplishment. With the semester coming to an end, it is necessary to look back and review these works and to fully recognize the importance of some of the greatest names in medical science.
The first Nobel Prize in Physiology or Medicine was awarded to Emil Adolf von Behring in 1901 for his discovery of blood serum therapy as a way to treat diphtheria [1]. During Behring’s time, diphtheria was a lethal disease but, as chronicled in Microbe Hunters by Paul de Kruif, no one had been able to isolate the agent causing the disease. It was eventually discovered that toxin secreted by the diphtheria microbe was causing the illness, so Behring used this information to study infected guinea pigs in Germany in the late 1800s (De Kruif, 1926). Behring noticed that when he took the blood serum from guinea pigs that survived injection of diphtheria and injected it into guinea pigs that were dying from diphtheria, they survived (De Kruif, 1926). Behring had successfully found the cure for diphtheria and as a result, the disease was no longer a death sentence. Today, diphtheria has been, for the most part, eliminated from developed countries and occurs in very small numbers elsewhere. The discovery of blood serum therapy as a way to treat disease saved millions of lives and is still used today, most recently as a treatment for the Ebola virus.
Eleven years later in 1912, Alexis Carrel won the Nobel Prize for his work involving the transplantation of blood vessels from one species to another [1]. Using male and female cats as his model organism, Carrel successfully transplanted blood vessels of a canine heart into a feline heart and monitored the animals for a short time before sacrificing them and analyzing them (Carrel, 1907). Previous attempts at transplants of this sort had failed, but Carrel understood the value of being able to transplant organs from one species into another. His work provided the basis of later transplant experiments, including the experiments performed by Joseph Murray involving kidney transplants which won the Nobel Prize in Physiology or Medicine 78 years later in 1990.
In 1923, the youngest person ever to win the Nobel Prize in Physiology or Medicine, Frederick Banting, won the award for his discovery of insulin [1]. While studying diabetic patients in Canada, he discovered that a pancreatic extract (now known as insulin) dramatically improved the condition of patients suffering from severe diabetes (Banting & Best, 1922). Banting monitored the patients before, during, and after treatment with this extract and observed reductions of sugar in the urine (glycosuria) and blood sugar (Banting & Best, 1922). He also observed that his patients were no longer lethargic and tired and were full of energy. Banting’s discovery of insulin was immediately recognized as he won the Nobel Prize only one year after his paper, “Pancreatic extracts in the treatment of diabetes mellitus” was published.
Thomas Morgan received the Nobel Prize in 1933 for his discoveries involving chromosomes and heredity [1]. Morgan was the first to propose that genes are linear despite the conclusion made by Castle that they were not (Morgan et al., 1920). Using Drosophila as his model organism, Morgan was also able to determine that some genes were sex-linked and were limited to the sex chromosomes (Morgan, 1910). Morgan’s work on genetics using Drosophila provided the ground work for Hermann Joseph Muller’s 1946 Nobel Prize for his discovery that x-ray irradiation can produce mutations [1]. At the time that Morgan and Muller were performing their genetics research, Barbara McClintock was also investigating genetics and heredity using corn as her model. Through studying the pigmentation of corn kernels, McClintock was able to discover mobile genetic elements for which she did not win the Nobel Prize until 1983 [1].
In 1929, Sir Alexander Fleming noticed that a contaminating Penicillium mold was inhibiting the growth of Staphylococcus colonies and was causing them to undergo lysis (Fleming, 1929). From this “by chance” observation, he had discovered penicillin, one of the most effective antibiotics. For this discovery, he received the Nobel Prize in 1945 along with Ernst Boris Chain and Sir Howard Walter Florey [1]. The discovery of penicillin had dramatic effects worldwide as it was able to treat a wide range of bacterial infections by inhibiting bacterial cell wall formation. Fleming’s work played a role in Selman Waksman’s discovery of streptomycin, which won him the Nobel Prize in 1952 [1].
The 1962 Nobel Prize in Physiology or Medicine went to James Watson, Francis Crick and Maurice Wilkins for their discovery of the molecular structure of DNA (the double helix) [1]. During the 1950s, the race was on to determine the structure of DNA and prominent scientists, including Linus Pauling, were actively searching for it. X-ray crystallographer Rosalind Franklin successfully produced images of DNA that clearly showed a helical pattern but without her permission, Watson and Crick used these images to propose the double helix structure in their 1953 paper “Molecular structure of nucleic acids: a structure for deoxyribose nucleic acid”. While we know now that Watson and Crick were correct, one of the most important parts of their paper comes in one of the last sentences in which they suggest that this structure may play a role in the copying of genetic information, which we also now know to be true.
The concept of the DNA double helix seems so basic to us today, but 60 years ago it was completely unknown. Another concept of biology that seems elementary to us, the organelles of the cell, was also not completely nailed down until recently. In 1974, George Palade received the Nobel Prize for discoveries regarding the structure and organization of the cell [1]. More notably, Palade discovered the ribosome; he noticed a “small granule” that was present in multiple cell types that seemed to concentrate around the endoplasmic reticulum, while a small minority could be found floating freely around the cell (Palade, 1955). Because of Palade’s discovery, we now understand the process of protein synthesis via translation within the cytoplasm of the cell.
In the 1980s, two very important Nobel Prizes were awarded in the fields of cholesterol metabolism and neuroembryology. In 1985, Michael Brown and Joseph Goldstein won the Prize for their discoveries involving cholesterol metabolism. Through studying a man with familial hypercholesterolemia, they were able to determine the mechanism of LDL binding in cells, the importance of coated pits on the cell surface, and how FH forms as a result of failure of the cells to uptake LDL (Anderson, Goldstein, & Brown, 1977). The following year, Rita Levi-Montalcini won the Prize for her discovery of growth factors. Using chicken embryos as her model organism, she exposed the embryos to mouse sarcomas and snake venom and noticed a dramatic increase in sensory ganglia growth (Levi-Montalcini & Cohen, 1956). From her experiments, she was able to determine that an agent secreted from the sarcomas and snake venom was causing the increase in nerve cell growth and today, this knowledge has been applied to cancer research.
Clearly, the awards mentioned in this review only summarize a few of the over 100 awards granted in the Physiology or Medicine category of the Nobel Prize. However, it is important to track the progress of the Prize as a way of understand how far we have come in science. When the Nobel Prize was first awarded, fields such as biochemistry, neuroembryology, and microbiology did not exist. But with advances in technology and understanding of basic cell processes, the works of Nobel Laureates has branched out to almost infinite scientific fields. The works by the scientists mentioned above have had profound effects on our society whether we realize it or not and without these discoveries, it is hard to visualize where we would be today.
References
[1] (2014). “All Nobel Prizes in Physiology or Medicine”. Nobelprize. org. Accessed 7 Dec 2014 from: http://www.nobelprize.org/ nobel_prizes/medicine/laureates/.
Anderson, R., Goldstein, J., & Brown, M. (1977). A mutation that impairs the ability of lipoprotein receptors to localize in coated pits on the cell surface of human fibroblasts. Nature, 270: 695-699.
Banting, F. & Best, C. H. (1922). Pancreatic extracts iin the treatment of diabetes mellitus. The Canadian Medical Association Journal, 141-146.
Carrel, A. (1907). Heterotransplantation of blood vessels preserved in cold storage. J. Exp. Med., 9(2): 226-228.
De Kruif, P. (1926). Microbe Hunters. San Diego: Harcourt, Inc.
Fleming, A. (1929). On the antibacterial action of cultures of a penicilli- um, with special reference to their use in the isolation of B. influenza. British Journal of Experimental Pathology, 10 (2): 226-236.
Levi-Montalcini, R. & Cohen, S. (1956). In vitro and in vivo effects of a nerve growth stimulating agent isolated from snake venom. PNAS, 42(9): 695-699.
Morgan, et al. (1920). The evidence for the linear order of the genes. PNAS, 162-164.
Morgan, T. H. (1910). Sex limited inheritance in Drosophila. Science, 32 (812): 120-122.
Palade, G. (1955). A small particulate component of the cytoplasm. J. Biophysic. And Biochem. Cytol, 1(1): 59-67.
Watson, J.& Crick, F. (1953). Molecular structure of nucleic acids: a structure for deoxyribose nucleic acids. Nature, 4356: 737- 738.