Methods of Gene Replacement Therapy in Mouse and Sheep Models of Tay Sachs Disease
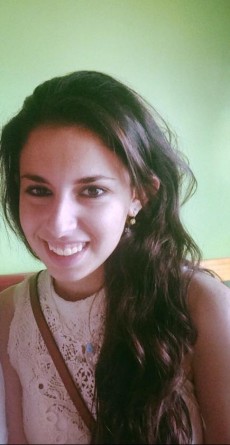
Sarah Chiren, Scott Johnston, & Morgan Marshall
Department of Biology
Lake Forest College
Lake Forest, IL 60045
Abstract
Tay Sachs Disease is a neurodegenerative autosomal recessive disorder characterized by the mutation of the HexA gene, which codes for part of the β-hexosaminidase enzyme that degrades GM2 glycolipids (gangliosides). GM2 glycolipids accumulate in the lysosome which causes neural inflammation, a weakened immune system and eventually, early death. No cure for Tay Sachs Disease or other GM2 ganglioside diseases (such as Sandhoff disease) exists, and researchers have faced difficulties in finding safe and effective methods. In 2012, Cachon-Gonzalez et al. showed improved HexB function through replacement therapy in Sandhoff disease through the use of recombinant adeno viral vectors (rAAVs). In 2014, Matsuoko et al. found that N-glycan (NG) attached to HexA improved cellular uptake of HexA and restoration of normal function in Sandhoff mice. However, it remains unclear if these methods can be used effectively to replace HexA in Tay Sach’s Disease. We hypothesize that by using different approaches to gene replacement therapy via iPSCs and rAAVs, using the WT and Ng Hex A modifications, Tay Sachs disease symptoms and pathology will be rescued. Furthermore, these methods will provide greater insight into Tay Sachs pathology as well as improved treatments for other ganglioside diseases.
Introduction
Tay Sachs Disease is a rare, autosomal recessive neurodegenerative disorder (38). Approximately 1 in 320,000 individuals from the general population will get Tay Sachs Disease, and 1 in 250 from the general population carry a gene for the disease (19). Due to high rates of intra-marriage, Ashkenazi Jews, the Cajuns, French Canadians, and the Irish Americans are slightly more susceptible to carry the disease; 1 in 27-40 individuals from these groups carry the disease, and 1 out of 4,000 Ashkenazi Jews will be born with the disease (27).
In 1881, Konrad Sandhoff identified a cherry-red spot during an eye examination, which was one of the first indicators of gangliosidoses, the hallmark pathological finding of Tay Sachs (10). In addition to the cherry-red spot, juvenile symptoms of Tay Sach’s Disease include spasticity, reduced attentiveness, loss of motor skills, blindness, seizures, and inflammatory responses producing enlarged organs (the spleen and the liver) (14). Though rare, adult Tay Sachs disease has similar symptoms: muscle weakness, trouble walking, speech and swallowing difficulties, and urinary incontinence (27).
One of the main molecules associated with the symptoms of Tay Sachs disease is a ganglioside found in the nervous system that is involved in biological pathways (9). Gangliosides are found in cytoplasmic membranous bodies along with cholesterol and phospholipids (26) Gangliosides vary by the number of sialic acids linked onto the sugar chain. Monosialic gangliosides are divided into three classes based on chronological finding: GM1, GM2, and GM3 (9). In Tay Sachs disease, the GM2 ganglioside substrate accumulates (14).
Like most macromolecules in the cell, GM2 ganglioside is degraded at optimal performance levels (24). The lysosomal enzyme β-hexosaminidase is involved in the degradation of GM2. β-hexosaminidase is a dimeric protein consisting of α and β subunits that are encoded by the HEXA and HEXB genes, respectively (37). Isozymes Hex A, Hex B, and Hex S are composed of subunit formations α/β, β/β, and α/α respectively (11). The Hex A isozyme aids in the breakdown of GM2 gangliosides by hydrolyzing them into GM3 gangliosides, which allows further enzymatic degradation. In Tay Sachs disease, HexA is mutated (17), and as a re- sult, the α subunit on the Hex A isozyme is misfolded, which ultimately leads to the loss of β-hexosaminidase enzymatic function (17). Similarly, in Sandhoff disease, the HexB gene is mutated, ultimately leading to a loss of β-hexosaminidase function. This halts the breakdown of GM2 gangliosides, leading to a progressive build up in the cell (23 & 16).
When gangliosides accumulate and cannot be degraded as a result of the dysfunctional β-hexosaminidase, the lysosome stores the accumulation (25). This lysosomal storage leads to lysosomal swelling, and therefore neural inflammation. The ganglioside accumulation has been associated with apoptotic cell death (25 & 18) but the aggregates may have neuroprotective-like properties, and another factor may be the cause of thecell death.
Although there is no cure for Tay Sachs Disease, there are several current therapies that aim to restore enzymatic function in gangliosidoses. In 2012, Cachon-Gonzalez used recombinant adeno associated viral vectors to replace α and β subunits of β-hexosaminidase to restore function and in 2014 Matsuoko et al. found that N-glycan (Ng) attached to HexA improved cellular uptake of HexA and restoration of normal function in Sandhoff mice (13). Additionally, researchers recently discovered a new model to observe Tay Sachs Disease symptoms. Current models for Tay Sachs disease include transgenic Hex A -/-, which are optimal for mea- suring Hex A enzymatic activity and GM2 accumulation but do not show any physical symptoms (38 & 2). A newly discovered population of Jacob Sheep has naturally developed a variation of juvenile Tay Sachs disease that parallels with human Tay Sachs disease (31). These sheep are currently being used to study motor symptoms of Tay Sachs disease with AAV gene replacement therapy however there has been no significant change in symptoms (20 & 31).
While the researchers Cachon-Gonzalez and Matsuoko et al have shown success in HexB deficient models, they have not been shown in HexA deficient models. In addition, currently gene replacement therapy trails by Torres et al on Tay Sachs Jacob Sheep have shown only a slightly significant increase in life span and symptom improvement; however, they have not looked into using different techniques for gene replacement therapy. We hypothesize that using different approaches to gene therapy, such as induced pluripotent stem cells (iPSCs), recombinant adeno associated viral vectors (rAAVs), and N-glycan modification, will show significant rescue of pathology and behavior.
SIGNIFICANCE
Broader Relevance: Tay-Sachs is a debilitating neurodegenerative disease that primarily affects young children. Approximately 1 in 320,000 individuals from the general population will get Tay Sachs Disease, and 1 in 250 from the general population carry a gene for the disease (19). Currently, there is no known cure and forms of treatment are inefficient and fail to stop the progression of the disease. As a result, children with Tay Sach’s Disease die before the age of 5 (19). The findings in this proposal will aid in expanding our knowledge on three promising methods of treatment on Tay-Sachs, hopefully aiding in diminishing the symptoms or restoring normal conditions of Tay-Sachs patients in the future. Most importantly, this study will contribute to future studies on this disease and help to further the work that has already been tirelessly put forth.
Intellectual Merit: Within this proposal, we will be examining three methods of therapy that have potential in effective treatments of Tay-Sachs. We propose to explore the efficiency of ERT and GRT, iPSC treatment and viral-vector treatment and compare their abilities to restore normal function in Tay-Sachs mouse models. This research could advance our abilities in finding proper pharmacological treatment of Tay-Sachs. Furthermore, this research can also open up more options for treatment in other lysomal storage disorders and other forms of GM2 gangliosidoses.
SPECIFIC AIMS
The end goal of this experiment is to compare and improve upon techniques that will help progress gene replacement therapy as a future tool to aid in Tay Sachs disease prevention. This study will also examine juvenile behavioral symptoms by using the newly discovered Jacob Sheep model.
1. Gene replacement therapy via pluripotent steam cell (iPSC) using WT correction: Induced Pluripotent stem cells will be differentiated into dopa- minergic and motor neurons using iPSC technology with the corrected WT HEXA gene. Cells will then be transplanted into both transgenic Hex A -/- mice and Jacob sheep. Hex A enzyme activity and GM2 accumulation will be tested for both model to see if there is any improvement upon pathology. Motor functions will be tested in Jacob sheep only because Transgenic Hex A -/- mice do not show physical symptoms of Tay Sachs disease.
2. Gene replacement therapy via rAAV using WT correction: Recombinant adeno-associated viral vectors containing α and β subunits of β-hexosaminidase and expressed in HEK293 cells. Cells will then be transplanted into both transgenic Hex A -/- mice and Jacob sheep. Hex A enzyme activi- ty and GM2 accumulation will be tested for both model to see if there is any improvement upon pathology. Motor functions will be tested in Jacob sheep because Transgenic Hex A -/- mice do not show physical symptoms of Tay Sachs disease.
3. Gene replacement therapy via rAAV and iPSC using NgHexA correction: Using both previously stated iPSC and rAAV methods, NgHexA gene will be used in place of the WT HEXA gene correction. The NgHex A gene will be transmitted into both Hex A -/- mice and Jacob sheep. Hex A enzymatic function along with GM2 accumulation will be measured to test upon any improvement. Motor functions of Jacob sheep will be tested using the step test and rotary test.
Research Designs and Methods
Aim 1: Use pluripotent stem cells to perform gene replacement therapy in a Tay-Sachs model.
Rationale: A previous study by Zou et al has shown successful gene correction of the mutated gene back to WT in sickle disease using induced pluripotent stem cells (iPSC) in cell culture (40). This study has shown evidence that iPSC can be used to successfully correct mutated genes back into the WT form for a specific disease. It is important to view this in the context of potential Tay Sachs disease therapy because iPSC have not yet been used for gene replacement therapy in Tay Sachs disease. Current GRT studies for TD only use adenovirus vectors (AAV) as a method for providing the wild type HEXA gene (5). However, this approach was unsuccessful after primate trials when unwanted symptoms arose after in- jection (20). By looking into possible restoration of WT Hex A via iPSC, a future therapy can be produced that helps improve upon the loss of Hex A enzymatic function, GM2 accumulation, and motor symptoms.
Design and Methods:
Making iPS cells into iNS cells: Cheek cells from the healthy WT mice will be taken and transformed into pluripotent state using iPSC technology (29 & 5). These cells will then be differentiating into various neural lineages, including dopaminergic neurons, and motor neurons (8 & 7). Differentiating neural cells will then be tested for if they exhibit the wanted characteristics of the listed neural linages using the method of immunocytochemistry (39). Staining of control motor and dopaminergic neurons will be compared to the experimental differentiated cells using a one-way ANOVA test for statistical significance. Prediction: iPS cells will display the characteristics of motor neurons and dopaminergic neurons similar to the control and statistically more than the undifferentiated negative control. If characteristics of wanted cells are not exhibited then the cells have not properly differentiated.
Transplanting iNS cells into mouse model and testing pathology changes: One control model will be acquired that has no alternations in DNA along with two experimental diseased models that express typical Tay Sachs pathology and behavioral symptoms. These models will be compared to each other with the non-diseased model being the positive control and the initial state of the diseased models being the negative control. When the diseased model begins to show symptoms of Tay-Sachs (around 3-4 weeks of age), neural cells will then be intergraded into various re- gions in the model’s brain using recently discovered successful transplant methods (6). Hex A enzymatic activity will be measured using 4-methy- lumbelliferyl-6-sulfo-N-acetyl-B-D-glucosaminde (MUGS) as a fluorogenic substrate (18). Relative GM2 accumulation within the models’ brains will be determined using electron microscopy to see if accumulation is present within multiple storage regions (18). Positive and negative controls for the Hex A activity and GM2 accumulation will be compared to the experimental models using a one-way ANOVA test for statistical significance. Prediction: After transplanting the WT HEXA gene into the diseased model, we expect to see increased levels of enzymatic Hex A activity along with a decrease in GM2 accumulation that resembles the control model’s natural levels. If results show something else, then gene therapy via iPSC was unsuccessful.
Transplanting iNS cells into Jacob Sheep and examining physical symptoms: If the previous method of transplanting iNS cells into a mouse model was successful, and Hex A activity increased, along with a decrease in GM2 accumulation, we will be able to move on to testing what these results mean for juvenile symptoms. Same procedure will be taken to create iPS and iNS cells and transplanting them into two diseased Jacob Sheep which naturally show the Tay Sachs HexA mutation (29, 5, & 7). Pre-inoculation, sheep’s motor functions will be examined with physiological function tests and then about 2-months post inoculation, motor functions will be examined again (31). Hex A activity and GM2 accumulation will also be examined using the previously mentioned methods (18). Healthy control Jacob sheep motor function and pre-inoculation stats will be compared against post-inoculation stats using a one-way ANOVA test for statistical significance. Predictions: There will be in increase in motor functions that correlates with increased Hex A enzymatic activity and de- creased GM2 accumulation. If there is no change in motor function, and enzyme activity does not change, then this approach was unsuccessful. If there was no change in motor function, but an increase in enzyme activity is seen, then this shows how Jacob sheep are not effective in studying juvenile symptoms.
Aim 2: To use viral-vector therapy to improve cell conditions and rescue normal cell function:
Rationale: Adenoviral and lentiviral vectors have been used in the past to express β-hexosaminidase but show little improvement in survival or functionality (21 & 30). In 2012, Cachon-Gonzalez et al. transfected the α and β subunits of β-hexosaminidase into a rAAV. When the rAAV was injected using ICV, HEXB deficient mice showed increased survival, decreased neural inflammation, an increased inflammatory response to lysosomal storage, and decreased storage (1). Because these results were successful in HexB deficient mice, it is important to test the method in Tay Sachs disease using HEXA deficient models to show application to Tay Sachs disease therapy.
Design and Method:
Obtaining model organisms: Jacob’s Sheep, which have a mutated HexA gene, can be obtained from the farm (31). These sheep will be used to test both pathology and behavior improvements. We will knock out the HexA gene from mice to test pathology and cell improvements (38). Mice will be provided cages and nutrients (1). A control wild type Hex A mouse will be acquired that has normal enzymatic function along with two Hex A -/- mice (negative controls). Healthy sheep will serve as a positive control while untreated Jacob Sheep will serve as a negative control.
Recombinant viral vector construction: To construct the rAAV, an AAV2 previral plasmid will be subcloned to contain the α and β subunit expression cassettes to direct RNA and protein production. The plasmid will be fused using cDNAs with RT-PCR, and HEK293 cells will be used to express the genes. The HEK293 cells will be cotransfected with an adenovirus helper plasmid and a chimeric packaging construct expressing the AAV2 rep gene and AAV2 cap genes. The viruses will then be purified by affinity column chromatography (41).
Inject rAAV and verify subunits: One-month-old HexA deficient mice will be injected using ICV (as explained in Aim 1) with rAAV expressing α and β subunits and sacrificed four weeks postinjection. To verify that the subunits are expressed in the mice, brain extracts from the striatum and cerebrum (1) will be analyzed using immunoblot analysis with goat antihuman antibody against HexA as well as fluorescence microscopy staining fluorescein for the α subunit and cyanine-3 to stain for the β sub- unit (21). Staining will be quantified and α and β subunit will be compared to controls (WT mice and untreated HexA deficient mice) using one-way ANOVA. Prediction: A significant increase in α and β subunit in treated mice compared to untreated mice. If there is no significant increase, the α and β subunits were not properly inserted into the vector, not properly expressed in the HEK293 cells, or not properly injected into the mice.
Test accumulation and inflammatory response: Cerebrum and striatum extracts will be stained for accumulation using periodic acid Schiff (PAS) reagent to test for glycoprotein accumulation (1). PAS staining will be quantified and compared to controls (WT mice and untreated HexA de- ficient mice) using one-way ANOVA. Prediction: There will be a significant decrease in glycolipid accumulation in rAAV treated mice than in untreated. If there is no significant decrease, the vector has not restored function of β-hexosaminidase. An indication of a rescue response to accumulation is the neural inflammatory response. To test for inflammatory response, cerebrum and striatum extracts will be stained using CD68 and GFAP to test for glial and astrocyte response, respectively. Amount of CD68 and GFAP cells staining will be quantified and will be compared to controls (WT and untreated HexA deficient mice) using one-way ANOVA. Prediction: There will be a significant increase in the glial and astrocyte response in treated mice when compared to untreated. If there is no increase in inflammatory response, cells may not be degrading glycoprotein.
Behavior observations: Jacob Sheep will be utilized to study improvement of motor function in response to rAAV therapy. A threshold of sheep’s motor functions will be taken using a rotary test and step test and used as a comparison. Two months post therapy; sheep’s motor functions will be examined again using the rotary test and step test. In a successful gene replacement, the sheep will show significantly improved rotary test and step test compared to the untreated group and compared to its threshold. Prediction: the sheep received both rAAV replacement will spend sig- nificantly longer on the treadmill in the rotary test than control sheep who have not received rAAV therapy and will also take significantly less time to walk up the stairs than control sheep who have not received the rAAV therapy. If sheep have not improved from the threshold, then the glycoprotein may not be fully degraded by β-hexosaminidase, or other pathology may contribute to the TSD symptoms.
Aim 3: Gene replacement therapy via rAAV and iPSC using Ng- HexA correction
Rationale: A previous study by Matsuoka et al. has shown that attaching an N-glycan to the alpha subunit of β-hexosaminidase improves upon its enzymatic function (13). Investigating this Hex mutation (Ng HexA) in the light of possible treatment for Tay-Sachs disease is important because it could combat the GM2 accumulation along with the loss of Hex A enzy- matic function that plays a significant role in the pathology of the disease (12). The effectiveness of pathological rescue between the WT Hex A and Ng Hex A therapies will be assessed to determine if Ng Hex A has a more enhanced effect than the wild type.
Design and Method:
Observe uptake of NgHexA and WTHexA within Tay-Sachs mouse and Jacob’s sheep model: A control wild type Hex A mouse will be acquired that has normal enzymatic function along with two Hex A -/- mice (negative controls). Healthy sheep will serve as a positive control while untreated Jacob Sheep will serve as a negative control. The mutated Hex A, Ng Hex A, will be achieved by using the method of CHO cell lines co-expressing the WT HEXB and genetically altered HEXA gene with an addition N-glycosylation consensus sequon (13). The uptake of WT HexA and Ng HexA in the experimental mouse and sheep models will be measured by using glycosylation analysis assay (13). Healthy positive control and Hex A -/- negative control will be compared to experimental models using a one-way ANOVA test for statistical significance Prediction: Models administered the Ng Hex A gene are expected to have statistically higher uptake level of both the healthy control and experimental models showing that Ng Hex A is received better than WT Hex A. If there is no significant different between uptake in Ng Hex A and WT Hex A then it can be concluded that Ng Hex A is received by the models just as well as the WT. If Ng Hex A has less uptake than the WT Hex A, then it can be concluded that Ng Hex A is not received better than WT.
Observe and compare WT HexA and NgHexA on GM2 accu- mulation and Hex A enzymatic function: Using the previously stated meth- ods of MUGs fluorogenic substrate assay and electron microscopy as described in aim one, Hex A enzymatic function and GM2 accumulation will be measured, respectively (17). Healthy positive control and Hex A -/- negative control fluorogenic substrate will be quantified and compared to experimental models using a one-way ANOVA test to see statistical significance. Prediction: We expect to see an increase in Hex A enzymatic activity and decrease in GM2 accumulation for both the WT and Ng Hex A groups with the Ng Hex A group having a greater rescue in comparison to the WT. If there is no significant difference between the WT and Ng Hex A rescuethen it can be concluded that Ng Hex A gene replacement therapy is no different than WT. If Ng Hex A rescue is less than WT rescue then it can be concluded that Ng Hex A for gene replacement therapy is not a good method for improving upon Tay Sachs pathology.
Behavior observations: Jacob Sheep will be utilized to study improvement of motor function in response to NG HexA therapy. As previously stated, a threshold of sheep’s motor functions will be taken using a rotary test and step test and used as a comparison (31). Two months post-injection, sheep’s motor functions will be examined again using a rotary test and step test. Healthy control Jacob sheep motor function and pre-innoculation stats will be compared against post-innocolation stats using a one-way anova test for statistical significance. Prediction: Sheep who have received the Ng HexA replacement will spend significantly longer time on the treadmill in the rotary test than control sheep that have not received NG HexA therapy and will also take significantly less time to walk up the stairs than control sheep who have not received the NG HexA therapy. If this is not seen then Ng Hex A replacement has no significant impact on motor symptoms of Tay Sachs disease.