Genetics and Cancer: Clonal Evolution in Fanconi Anemia
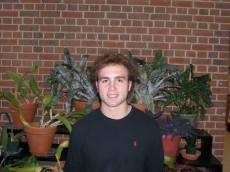
Christian Athens
Department of Biology
Lake Forest College
Download PDF
Abstract
Cancer has been known to evolve at an increased rate in individuals with a mutation or mutations to genes that function in DNA repair. A mutation of this sort can cause genetic instability and an increased mutation rate, both of which would contribute to the evolution of cancer. Studying diseases with similar mutations can provide us a more thorough understanding in the pathogenesis of cancer. A rare genetic disorder, known as Fanconi anemia, is believed to be the result of mutations to genes involved in DNA repair and protecting hematopoietic stem cells. Individuals with the disease many times experience bone marrow failure and an elevated risk of certain cancers such as acute myeloid leukemia. The evolution of cancer in these individuals can be explained through a process similar to natural selection but at the cellular level. Insights from journal articles and research done on Fanconi anemia and the bone marrow failure and cancer that many times result are used as a basis for this paper.
Cancer is a major concern for many people around the world, especially for people with a predisposition to cancer. Certain cancers are believed to evolve at an increased rate in individuals who possess a mutation in a gene or genes that function in DNA repair1. Mutations in DNA repair genes will allow for increased mutation rates in certain cells because the mutation leaves them genetically unstable.1 Normal body cells grow, replicate, and die in an orderly fashion, but this is not the case with cancer. Cancer starts when cells in a certain area of the body begin to grow out of control. Cancer evolves from a single cell that accumulates mutations, which allow it to survive and replicate at a greater rate than normal neighboring cells1. The cell then divides, its offspring divide, and then their progeny divide and so on to produce a large population of abnormal cells, this is known as clonal evolution. The mutations that allow a cell increased growth and replication are usually either an inactivating mutation to a gene, such as a tumor suppressor gene, that inhibits or regulates cell growth, or one that activates a gene, known as an oncogene, that encourages cell growth and replication. A mutation of either kind would therefore greatly benefit a cell over other neighboring cells that have not experienced a mutation of this sort. Environmental factors such as diet, tobacco use, and exposure to carcinogens, as well as genetic factors such as inherited genetic disorders are all known to have a primary role in the evolution of cancer.1
Analyzing rare genetic diseases with a predisposition to cancer can be a valuable approach to gain insights into the pathogenesis of cancer. A rare bone marrow disorder known as Fanconi anemia (FA), frequently results in bone marrow failure and cancers such as, leukemia and solid tumors. Individuals with bone marrow disorders are at an increased risk for the development of clonal neoplasm’s, such as acute myeloid leukemia (AML)2.
According to an article by Lensch et al. “the prevalence of AML in FA is estimated to be 15,000 times greater than that of the general population.”3 Fanconi anemia is defined by an inactivating mutation to any of the 13 FA genes, whose function is believed to be in DNA repair and protecting hematopoietic stem cells.4 Given this, an inactivation of this gene is expressed through genetic instability and hypersensitivity of hematopoietic stem cells to apoptotic cues.4 Many individuals with FA who experience bone marrow failure also develop cancer. This high rate of clonal evolution in FA can be explained through a process analogous to natural selection, which is evoked by the genetic instability and cellular hypersensitivity characteristic to the disease. Insights from journal articles on bone marrow failure and clonal evolution in FA will help support this notion.
According to the theory of natural selection, there must be a variation in traits, differential reproduction and heritability of these traits for natural selection to act on a population. In an article published in 2007, the researchers’ Bagby and Meyers applied the process of natural selection to clonal evolution. They stated, “There must be a sufficiently high selection pressure, a stem cell population of sufficient size, and a significant mutation rate in order for clonal evolution to occur.”2 We will first begin with bone marrow failure when explaining this model because it plays a predominant role in the clonal evolution in FA.
Hematopoietic stem cells are cells found in the bone marrow and give rise to all types of blood cells. In the FA hematopoietic stem cell pools, these hypersensitive cells are exposed to cytokines that acts as cues for apoptosis, such as tumor necrosis factor-alpha (TNF-a). Bone marrow failure that occurs in FA is directly related to the hematopoietic stem cells hypersensitivity to apoptotic cues.2 Normal stem cells would be relatively resistant and maintain a sufficient population size, whereas the FA stem cells decrease in number due to the increased rate of apoptosis caused by the stem cell’s hypersensitivity to certain cytokines. Thus, the production of blood cells decreases and bone marrow failure results. So far we have two of the three requirements for clonal evolution: an initial stem cell population of sufficient size, as seen before bone marrow failure, and a strong selection pressure noted by the strong selection against the hypersensitive hematopoietic stem cells. The final requirement will be a result of the genetic instability characteristic to FA. The increased rate of mutation associated with genetic instability will likely lead to a cell that experiences a mutation or mutations that allow it resistance to the apoptotic cytokine. The effect of the FA mutation initially causes the hypersensitivity of the hematopoietic stem cells and then the genetic instability leads to a cytokine resistant clone.3
Putting these steps together will help create a clear picture of what causes clonal evolution in FA. First, we have the FA hematopoietic stem cells, which are hypersensitive to apoptotic cues and bone marrow failure usually follows. Next, because the stem cells are hypersensitive to the apoptotic cues there is strong selection for a cell resistant to these apoptotic cues. Then, a cytokine resistant clone emerges due to the high rate of mutation in the stem cells. Finally, because the adapted clones have a major competitive advantage, they continue to proliferate while the non-adapted hypersensitive cells are purged and clonal evolution occurs.2 In short, the FA hematopoietic stem cells’ hypersensitivity and increased rate of mutation in the pro-apoptotic environment favors the evolution of a cytokine resistant clone.
Next, the researchers transplanted WT cells and the TNF-a resistant Fancc-/- cells into mice in an attempt to determine whether the TNF-a resistant Fancc-/- would give rise to leukemia. Mice that received WT cells survived 150 days without developing leukemia, as we would have expected. The mice that received the TNF-a resistant Fancc-/- cells were not so fortunate. All of these mice developed leukemia within 150 days and the TNF-a resistant Fancc-/- cells accounted for more than 90% of their blood.4 These results have shown that the Fancc-/- cytokine hypersensitivity in the presence of TNF-a has accounted for the emergence of the leukemic TNF-a resistant Fancc-/- cells.
This study supports notion that the cytokine hypersensitivity and genetic instability expressed in the FA hematopoietic stem cells leads to rapid clonal evolution in FA. The high mutation rate in the FA hematopoietic stem cells increases the likelihood that a cytokine resistant clone will emerge and strong selection for this cytokine resistant clone allows for its proliferation and success over the non-adapted cells, which ultimately leads to clonal evolution. Given the nature of clonal evolution in FA, lowering the selection pressure in the hematopoietic stem cell’s microenvironment may help lower the chances of developing cancer or at least slow the rate at which it progresses. Understanding how cancers evolve in genetic diseases may allow physicians to treat the problem in its early stages or even before it arises by closely monitoring and/or introducing novel treatments to patients with genetic diseases that have a predisposition for certain types of cancers.
References
1. Gelehrter, Thomas D., Francis S. Collins, and David Ginsburg. “Cancer Genetics.” Principles of Medical Genetics. Second ed. Baltimore: Williams & Wilkins, 1998. 245-72.
2. Bagby, Grover C., and Gabrielle Meyers. “Bone Marrow Failure as a Risk Factor for Clonal Evolution: Prospects for Leukemia Prevention.” American Society of Hemotology (2007): 40-46.
3. Lensch, M. W., R. K. Rathbun, S. B. Olson, G. R. Jones, and G. C. Bagby. “Selective Pressure as an Essential Force in Molecular Evolution of Myeloid Leukemic Clones: a View from the Window of Fanconi Anemia.” Leukemia (1999): 1784-789.
4. Li, June, Daniel P. Sejas, Xiaoling Zhang, Yuhui Qiu, Kalpana J. Nattamai, Reena Rani, Keaney R. Rathbun, Hartmut Geiger, David A. Williams, Grover C. Bagby, and Qishen Pang. “TNF-a Induces Leukemic Clonal Evolution Ex Vivo in Fanconi Anemia Group C Murine Stem Cells.” Journal of Clinical Investigation 117.11 (2007): 3283-295.