Developing multiple model systems to elicit PANK2 rescue for PKAN patients
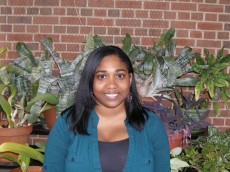
Department of Neuroscience
Lake Forest College
Lake Forest, Illinois 60045
Download PDF
Abstract
Pantothenate kinase-associated neurodegeneration (PKAN) is an autosomal recessive disorder characterized by iron accumulation in the brain. Neuropathology shows progressive loss of neurons and an increase in axonal swelling. PKAN is caused by mutations in the PANK2 gene found on the short arm of chromosome 20p13. It is estimated that 1 in 500 people carry a PANK2 mutation. The disease causing mutations in PANK2 has been linked to coenzyme A (CoA) metabolism. PANK2 localizes to mitochondria, suggesting a role in lipid homeostasis. It also codes for the mitochondrial enzyme, pantothenate kinase, which regulates pantothenic acid (vitamin B5). While the disease has rarely been studied, recent insights from mouse and Drosophila models of PKAN exhibit signs of decreased CoA activity and suggests that the PANK2 gene may have rescue properties if overexpressed or if pantothenic acid is added. This finding has led to our hypothesis that PANK2 or pantothenic acid will rescue PANK2 deficient neuronal cells and that CoA metabolism plays a crucial role in PKAN pathogenesis. To test our hypothesis, we aim to 1) generate a gene deletion of PANK2 in a mouse and feed it pantothenic acid deficient diets in addition to showing PANK2 rescue abilities, 2) to generate a yeast model for studying PANK2 deficient neurotoxicity and show a rescue by PANK2 and 3) to investigate the effect of PANK2 and pantothenic acid on human cell line cultures. We believe that these findings will stimulate new therapeutic techniques for the treatment of PKAN.
Background
The global prevalence of neurodegenerative diseases has doubled in recent times as life expectancy and population ages increase. However, there is an emergence of degeneration pathologies occurring in younger individuals as small as infants. The range of subcellular and molecular levels of functioning tends to put the many types of neurodegeneration at parallels, but often will have similar pathologies. Pantothenate kinase-associated neurodegeneration, previously known as Hallervorden-Spatz disease, occurs in children and is associated with iron accumulation in the brain (1).
Pantothenate kinase-associated neurodegeneration (PKAN) has its onset within the first ten years of development and follows a relentless progression that leads to death by early adult life. The prevalence of PKAN can typically be 1-3 in a million individuals, making it a very rare form of degeneration. The disease first presents itself with gait abnormalities and mental deterioration in the first few years of onset, and can gradually progress to loss of speech or blindness (1, 2, 3, 4, 5). Cases of PKAN show a progressive loss of motor function, dystonia, serve rigidity, dementia, and symptoms that overlap with Parkinsonism (3, 6). PKAN pathology is characterized with destructive lesions of the globus pallidus, a structure implicated in the regulation of voluntary movement (2, 3). PKAN is also characterized by lesions within the substantia nigra pars reticulate, as well as loss of myelinated fibers and neurons with gliosis (7, 8). One of the neuropathologic findings of a group of neurodegenerative disorders, namely PKAN, is the presence of axonal spheroids in the terminals and distal ends within the central nervous system. This indicates that this disorder could be one of axonal transport dysfunction and lipid metabolism interference (9, 10). T-star weighed MRIs highlight the characteristic microscopic feature of PKAN as clear granular pigments and brownish glial pigments (10). Post mortem studies revealed that there was a significant amount of pigment accumulation which was later shown to contain iron. It is for this fact that the disorder is also known as neurodegeneration with brain iron accumulation type 1 (NBIA-1).
PKAN is known to be an autosomal recessive disorder and thus both parents must be heterozygous carriers for the disease. The gene was discovered on the chromosomal locus 20p13 (11). A 7-base pair deletion in an Amish pedigree was observed in a novel pantothenate kinase gene, PANK2, confirming that this gene was responsible for PANK2 pathogenesis (12,13). Mutations for the PANK2 gene correlates with a loss of function associated with the disorder. The functional basis of PANK2 has been shown to be essential for coenzyme A (CoA) biosynthesis as well as playing a role in the phosphorylation of pantothenic acid (14). Amino acid analyses in the brain of patients with PANK2 show elevated levels of cysteine, which becomes consumed by the biosynthesis of CoA function of PANK2. It is believed that a loss of functional PANK2 accounts for the accumulation of cysteine in brain areas of this neurodegenerative disease (15). Cysteine is known to have multiple iron bonds which could potentially account for the noted iron accumulation found in PKAN patients. This accumulation accounts for an increase in toxicity as a result of oxidative and free radical damage (15, 16, 17).
Mutations of the PANK2 gene are thought to be a major contributing factor of PKAN pathology. There are about 100 mutations in PANK2, all missense, resulting in amino acid changes. For example, the most common PANK2 mutation Gly411Arg (G411R), which prevents cells from producing the functional enzyme pantothenate kinase (12,). Kuo et al. (2007) found that mice that were treated with pantothenic acid showed a significant reversal of symptoms, suggesting that the properties of pantothenate could have positive treatment effects for PKAN (18). Although a fly model has previously shown that pantothenic acid can rescue decreased CoA levels and rescue mitochondrial dysfunction, other models have not been implemented to show this phenomenon (19). Current models that try to define the specific phenotypic features of the disease due to a mutation in the PANK2 gene have been unsuccessful, and so it is still uncertain how an inactivation of PANK2 causes the specific molecular mechanistic changes observed in the disease. This leads to our gap in knowledge of whether we can create a variety of viable models for the phenotypic characteristics of the disease and thus rescue the loss of functional PANK2 by treating with pantothenic acid or overexpressing PANK2.
Therefore, we propose three new methods for testing rescue. The first model will be a mouse model in which the mice lack PANK2 and will be fed pantothenic acid deficient diets, as previously described (18). The second model will be a yeast model; the study of neurodegenerative diseases in yeast has recently become a very popular model source. Yeast can unravel complex mechanisms and pathways related to diseases and can expand our understanding of PKAN (20). As mentioned before, PANK2 gene mutations are known to cause PKAN. Both mice and Drosophila contain a homolog for the PANK2 gene found in humans. It has recently been documented that yeast also carry a homolog for PANK2, which is referred to as CAB1 in yeast (21). Lastly, human induced pluripotent stem cells (iPSCs) will be differentiated into neurons, the type of cell that is dying in PKAN patients. Diseased patient liver cells will be collected in order to create PANK2 mutated cells. Phenotypes such as iron accumulation, axonal swelling, and mitochondrial dysfunction will be observed. We will also take a look at the effect of PANK2 deficiency on CoA levels as this characteristic is well described throughout the neuropathology of the disease. All three models will be treated with pantothenic acid or PANK2 and we will observe whether these phenotypes can be recovered. We hypothesize that pantothenic acid and PANK2 will rescue PANK2 deficient or mutated neuronal cells, and that CoA metabolism plays a crucial role in PKAN pathogenesis. We believe that these findings will stimulate new therapy techniques for the treatment of PKAN.
Relevance
Broader Relevance: PKAN affects patients at a very young age, with very little chance of survival into adulthood. Although this disease affects 1 to 3 in one million people, the symptoms are quite devastating and severe. These patients affected by PKAN eventually die from loss of motor function, in which patients are unable to swallow or move any limbs, and the buildup of iron in the brain. Both the symptoms and pathogenesis of this disease are difficult to relieve and there is no effective treatment or cure. In that case, it is most crucial to study the disease in depth to produce effective treatments that will potentially delay the deadly symptoms of PKAN. Although it is rare and its discovery was made fairly recently, there is very little knowledge about its cause or what mechanisms in the body are being affected. Therefore, studying PKAN and its phenotypes in multiple organisms may reveal potential working therapies and treatments to help the young children who suffer from this ailment.
Intellectual Relevance: The central aim of this proposal is to study the mechanisms and phenotypes brought on by the PANK2 gene in three different model systems: mouse, yeast, and human cell cultures. This study will aid in discovering a therapy for PKAN patients. There are currently no significant treatments for PKAN and, as with most neurodegenerative diseases, treatments focus only on alleviating symptoms. This study will allow us to target the specific gene, PANK2, as means for eradicating the disease. Conducting research for the disease in various models will allow us to understand how the PANK2 gene is conserved and what functions or mechanisms are disturbed by ridding the model of this gene or by observing the effects of its mutations. The disease has never been expressed in a genetic model system such as yeast, which has a PANK2 gene homolog named CAB1, but we do know the powerful intrinsic information we can obtain from such diverse model systems which this proposal addresses. We hope that this study will facilitate in the advancement and understanding of this relatively unknown disease and will inspire researchers to study it more in depth to discover more effective treatments.
Specific Aims
The aim of our proposal is to replicate a gene deletion of PANK2 in a mouse model (18), as well as to generate a gene deletion of PANK2 in a yeast model, and human cell cultures. We hypothesize that depleted or mutated PANK2 will bring about PKAN pathophysiology, and the addition of pantothenic acid and PANK2 will rescue PKAN phenotypes. These various models will expand the knowledge of how the PANK2 gene functions and whether pantothenic acid and PANK2 are promising therapeutic agents for PKAN.
1. Evaluate the genetic rescue of PANK2 in a mouse model: A PANK2 (-/-) mouse model will be made by deleting the PANK2 gene from the cell line. The mice will be fed pantothenic acid deficient diets to ensure that the PANK2 gene is completely gone. We will evaluate the following pathologies: iron accumulation, axonal swelling, and mitochondrial dysfunction.
2. Establish a yeast model for PKAN: The yeast model will be created by using cell lines with deletion of CAB1 and evaluating the following pathologies: iron accumulation, decreased CoA levels, and mitochondrial dysfunction. Genetic and chemical rescue of PANK2 in yeast by overexpressing wild-type PANK2 will be evaluated.
3. Establish human iPSC cultures for PKAN and evaluate chemical/genetic rescue: Both PKAN patient and normal patient liver cells will be collected. Using an iPSC method, neurons will be generated and used to observe genetic and chemical rescue throughout neuronal cells. Iron accumulation, axonal swelling, and mitochondrial dysfunction will be studied to assess rescue.
Research Methods and Design
1. Evaluate the genetic rescue of PANK2 in a mouse model
Rationale: PANK2 knockout mice typically do not show the phenotypic characteristics of the disorder, but when they are fed a pantothenic acid-deficient diet, phenotypes are observed. Therefore, we want to replicate the experimental techniques used by Kuo et al (2007) to develop a PANK2 knockout mouse model for PKAN. The paper showed that pantothenic acid is the substrate for the enzyme pantothenate kinase 2 (PANK2) and highlighted its importance/ primacy in PKAN functioning. Mechanisms targeting the pantothenic acid component of the enzyme rather than the gene itself will allow us to model the pathological features of PKAN in our mouse model. We hypothesize that a pantothenate deficient diet will result in the hallmark pathologies of PKAN that is characteristic of iron accumulation, axonal swelling, and mitochondrial dysfunction which will be higher in the PANK2 knockout mice compared to wild type mice. Both juvenile and adult males will be used to compare the progression of the disease at different age groups and developmental stages.
1. Generation of the mouse knockout model: A group of 10 juvenile and 10 adult male mice will be used in this study. To develop our mouse model for PKAN, we will replicate the cre-lox recombination methodology (18) to remove the PANK2 gene. Insertions of cDNA containing thymidine kinase, neomycin resistance, and a loxP site will be made in between exons 2 and 3 of the PANK2 gene. A targeting vector created from cloning fragments of a mouse PANK2 genomic DNA will be inserted in the embryonic stem cells of a mouse strain (22). To validate this, we will obtain a viable model from the cre-recombinase mediated deletion. We will determine whether the knockout allele lacks the exon 3 as well as confirming that there was a germline transmission of the modified PANK2 gene in chimeric pups. To confirm PANK2 deficiency in the knockout mice (KO) allele, a RT-PCR analysis will be run to determine PANK2 expression. To also increase the validity of our PANK2 (-/-) model, these mice will also be fed pantothenic-acid deficient diets. By feeding the wild type (+/+) and PANK2 (-/-) mice a diet that is mixed with or without pantothenic-acid, we will be able to confirm and observe the genetic changes by using PCR amplification of the genomic DNA (18). These mice will be compared to non-transgenic mice, which is the negative control.
Next we created three assays to measure changes in brain pathology, indicative of iron accumulation, axonal swelling and mitochondrial dysfunction. Iron accumulation will be seen using immunohistochemistry with a cysteine-specific antibody, given that cysteine is bound to iron (15, 16, 17). Also, axonal swelling will be measured by taking a photomicrograph image (23) and will be quantified by measuring the axon per day. Mitochondrial dysfunction will be observed using a J-aggregate-forming lipophilic cationic (JC-1) assay with immunofluorescence and will be quantified, as described previously (19). Another method utilized will be an electron micrograph image revealing destroyed mitochondria. We will also characterize the known dysfunctions associated with PKAN, such as movement, body weight and poor grooming to determine whether the full range of the disorder was truly captured (symptoms and pathology).All will be quantified and compared to normal mice. Furthermore, mice will perform a rotorod test and will be placed in an open area to observe abnormalities in locomotion. This will determine the total motor activity of knockout mice compared to wild-type mice.
Prediction: We predict that the PANK2 (-/-) chimeras will exhibit these pathological characteristics: iron accumulation, axonal swelling, and mitochondrial dysfunction, as well as display a disruption in movement ability. We also predict that these mice will have a short life span. The pantothenic-acid deficient diets will elicit the neurological manifestations of PKAN and these mice will perform poorly on the motor-type tasks. If the knockout mice do not exhibit any of the known pathologies and movement disabilities, then this suggests that PANK2 (-/-) is not a sufficient model of PKAN.
2. Determination of the PANK2 rescue effects on the knockout mice: To determine whether pantothenic-acid is able to rescue the genetic changes that are brought on by a pantothenic-acid deficient diet mouse model, we will re-add pantothenic acid into the diets of our current model. Restoring pantothenate levels will allow us to determine and compare what changes occurred with varying levels of pantothenic-acid within the brain.
Prediction: We predict that a restoration of pantothenate in the diets of the knockout mice will lead to a recovery of the observed neuropathological characteristics and movement abnormalities. If there is no difference between the pantothenic acid deficient diets and the pantothenate acid rich diets in terms of restoring changes in pathology, then this suggests that pantothenic acid is not an adequate rescue model for the study of PKAN.
2. Establish a yeast model for PKAN
Rationale: The range of model systems for PKAN is limited to animal systems. A PANK2 gene has been located in yeast and is known as CAB1. Yeast is an excellent model system as it is easy to manipulate and culture in laboratory settings. We will generate a methodology from knowledge learned in a Cell and Molecular Biology course and use polymerase chain reaction (PCR) techniques to create a yeast model that has knockout PANK2 and compare it to a negative control yeast culture. We hypothesize that we will be able to see the pathologies associated with PKAN, such as iron accumulation, and that we can elicit a rescue response of PANK2 in the yeast knockout model.
1. Generation of the yeast knockout model: To create our yeast model, we will use PCR with forward and reverse primers that will exclude the PANK2 gene. By creating a PCR fragment that will flank the PANK2 homolog, CAB1, we will be able to obtain a PCR product that can be transfected into the yeast model, saccharomyces cerevisiae. The CAB1/PANK2 primer will allow us to generate a colony of yeast that lacks CAB1/PANK2. To confirm that the CAB1 gene has been deleted, we will use colony PCR for analysis. According to Roualt et al (2001), cysteine accumulation appears to have a direct role in iron accumulation and so we will determine whether there is an accumulation of iron indicated by cysteine expression, lipid formation, and a reduction of CoA levels present in the yeast strain due to a loss of the PANK2 gene. Cysteine and iron accumulation will be observed using a cysteine-specific antibody using the technique of immunohistochemistry. Lipid formation will be observed by using a lipophylic dye Nile red and using electron microscopy to demonstrate lipid accumulation (23). CoA levels will be observed using a HPLC methodology, as previously described (19). The yeast cells will be centrifuged with various buffers and the supernatant will be collected for HPLC analysis of CoA levels (19). Each of the 20 trials we intend to use will be quantified for cell growth and longevity.
Prediction: We expect to see increased iron accumulation, increased lipid formation, and decreased levels of CoA when compared to normal yeast cells. We also predict that Yeast cell death may increase in the CAB1/PANK2 lacking cells. This will translate into the CAB1/PANK2 phenotype and will be ready for pantothenate rescue. If these characteristics are not seen, then we would assume that yeast is not a good model for studying PKAN disease and that more research must be done to see whether other factors may be more important to study.
2. Rescue in the yeast knockout model: In order to evaluate rescue, we will insert the cell with the CAB1/PANK2 gene using a viral vector. We will use immunoblot analysis of the cells expressing the CAB1-GFPs, which will verify its presence. Cysteine-iron accumulation will be observed using a cysteine-specific antibody using the technique of immunohistochemistry. Lipid formation will be observed by using a lipophylic dye Nile red and using electron microscopy to demonstrate lipid accumulation (23). CoA levels will be observed using a HPLC methodology, as previously described (19). The yeast cells will be centrifuged with various buffers and the supernatant will be collected for HPLC analysis of CoA (19).
Prediction: We expect to see that the CAB1/PANK2 gene insertion will decrease the amount of cysteine-iron accumulation, lipid formation, and increase CoA levels. If the data suggest such a finding, then this model will be seen as ideal for the study of PKAN disease. If either of these characteristics are not observed, then the model will need to be modified for future use.
3. Establish human iPSC cultures for PKAN and evaluate chemical and genetic rescue.
Rationale: Studying PKAN in induced pluripotent stem cells (iPSCs) will broaden our understanding of how this disease works in human cells. Since they will be derived from the patient’s own cells, the body should accept these cells as their own. Previous findings have shown that iPSCs are useful in studying a variety of neurodegenerative diseases as well as new therapies that can change the lives of diseased patients (24, 25, 26, 27, 28). This method will provide a way to model the disease with no ethical dilemmas, unlike embryonic stem cells, yet are very similar. We hope to use pantothenic acid and PANK2 as a therapy for the mutated PANK2 cells derived from the PKAN patients in order to rescue its normal phenotypes, as described previously (19). iPSCs are most beneficial for drug therapy and discovery because it will increase the efficiency of discovering novel, effective drugs. An ANOVA test will be used to observe variance in the three variables tested in this proposal: iron accumulation, axonal swelling, and mitochondrial dysfunction.
1. Creating human-derived induced pluripotent stem cells (iPSCs): First, a liver biopsy will be conducted to collect liver cells from both normal study patients, which will be the negative control, and PKAN patients. We will introduce a set of transcription factors linked to pluripotency that will reprogram these cells into iPSCs. The most often used reprogramming factors include OCT4, SOX2, and KLF4, yet recent findings have proven that OCT4 is enough to create the neuronal stem cells that we are looking to create (29). Therefore, we will use this approach to create our diseased and normal neurons. The cells will be plated on feeder cells for four to six weeks. After the colonies have formed, we will select the colonies that are best fit to study, mainly those that have fully developed and lack tumor-like cells. The colonies will be then expanded, up to six germ lines, and frozen, which takes about six to eight weeks. STEM diff™ Neural Induction Medium will be used to differentiate the pluripotent cells into neural progenitor cells (NPCs), and such formation can be confirmed when neural tube-like rosette structures are seen in an immunofluorescence test using NPC markers such as PAX6, SOX1, and Nestin (30, 31). At this point, we will have differentiated neural cells from normal and diseased patients, which mean that the normal patient-derived cells will contain normal PANK2 while the other, diseased patient-derived cells will contain mutated PANK2. Iron accumulation will be observed through immunohistochemistry using a cysteine-specific antibody. Also, axonal swelling will be seen by using a photomicrograph image (23) and will be quantified by measuring the axon per day. Additionally, mitochondrial dysfunction will be observed using J-aggregate-forming lipophilic cationic (JC-1) assay with immunofluorescence and will be quantified, as described previously (19). Another method utilized will be an electron micrograph image revealing destroyed mitochondria, rendering it dysfunctional. All tests will be conducted four times to validate accurate findings. Rapid cell death may be an effect of the PANK2 mutation. Therefore, we will calculate longevity of the cells and count the number of resulting colonies per day. An ANOVA test will be utilized to study statistics.
Prediction: If the iPS neurons from the PKAN patient neurons exhibit increased iron accumulation, axonal swelling, and mitochondrial dysfunction compared to the normal patient iPS neurons, we can assume that this model is ideal for studying the disease and future potential treatments. If the cells exhibit less than all three of these characteristics, or at varying degrees compared to PKAN patients, then we would conclude that this model is not ideal for studying PKAN, yet it can still be used to study the cause for those characteristics in the disease.
2. Addition of pantothenic acid and overexpressing PANK2: A plasma or viral vector containing the pantothenic acid will be added into the neurons with mutated PANK2, the iPSCs created from a PKAN patient. PANK2 will be injected into a second colony to observe these results as well. In order to assess rescue, iron accumulation will be observed through immunohistochemistry using a cysteine-specific antibody. Also, axonal swelling will be seen by taking a photomicrograph image (23) and will be quantified by measuring the axon per day. Additionally, mitochondrial dysfunction will be observed using J-aggregate-forming lipophilic cationic (JC-1) assay with immunofluorescence and will be quantified, as described previously (19). Another method utilized will be an electron micrograph image revealing destroyed mitochondria. As with our previous proposed experiments all tests will be ran four times to validate accurate findings and comparisons will be made to the neurons with normal PANK2, the iPSCs created from a normal study patient. Again, rapid cell death may be an effect of the PANK2 mutation. Therefore, we will calculate longevity of the cells and count the number of resulting colonies per day. An ANOVA test will be utilized to study statistics.
Prediction: If the neurons from the diseased patient that are treated with pantothenic acid or PANK2 exhibit decreased iron accumulation, decreased mitochondrial dysfunction, and decreased axonal swelling when compared to the neurons from the diseased patient that are not treated with pantothenic acid or PANK2, then this rescue will serve as an excellent therapeutic drug that will reverse the PANK2 mutated phenotypes. This success may also account for the step towards clinical trials in human PKAN patients. If the neurons exhibit less than all three predicted characteristics, or an opposite, stagnant or increased levels of a characteristic, this model will not be considered to exhibit rescue and will therefore be reevaluated to better understand why the same or opposite effects are seen.
Note: Eukaryon is published by students at Lake Forest College, who are solely responsible for its content. The views expressed in Eukaryon do not necessarily reflect those of the College. Articles published within Eukaryon should not be cited in bibliographies. Material contained herein should be treated as personal communication and should be cited as such only with the consent of the author.
References
- Parmar A, Khare S, Srivastav V.J (2012) Pantothenate - kinase associated neurodegeneration. Assoc Physicians India. 2012 Apr; 60:74-6
- Donaldson, Ivan/ Marsden, C. David/ Schneider, Susanne A./ Bhatia, Kailash P (2012) Marsden’s Book of Movement Disorders (CH 11:PKAN, previously also known as Hallervorden-Spatz disease)
- Dooling, E.C, Richardson EP Jr., Davis K.R (1974) Hallervorden-Spatz Syndrome. Archives of Neurology 30:70-83
- Schneider SA, Hardy J, Bhatia KP. (2012) Syndromes of neurodegeneration with brain iron accumulation (NBIA): an update on clinical presentations, histological and genetic underpinnings, and treatment considerations. Mov Disord. 27(1):42-53
- Rossi, Daniela (2012) Early-onset neurodegeneration with brain iron accumulation due to PANK2 mutation. Brain & development vol:34 iss:6 pg:536 -538
- Hartig, B (2012) Pantothenate - kinase associated neurodegeneration. Current drug targets. M vol:13 iss:9 pg:1182
- Frederik CLEMENT, David DEVOSCaroline MOREAUPhilippe COUBESAlain DESTEE and Luc DEFEBVRE (2007) Neurodegeneration with brain iron accumulation: clinical, radiographic and genetic heterogeneity and corresponding therapeutic options.. Acta neurol. belg 107, 26-31
- Brunetti D, Dusi S, Morbin M, Uggetti A, Moda F, D’Amato I, Giordano C, d’Amati G, Cozzi A, Levi S, Hayflick S, Tiranti V(2012). Pantothenate kinase-associated neurodegeneration: altered mitochondria membrane potential and defective respiration in Pank2 knock-out mouse model. Hum Mol Genet.
- Dezortova M, Herynek V, Krssak M, Kronerwetter C, Trattnig S, Hajek M. (2012) Two forms of iron as an intrinsic contrast agent in the basal ganglia of PKAN patients. Contrast Media Mol Imaging 7(6):509-15.
- Rouault T.A., (2001) Iron on the brain. Nature Genetics 28:299-300
- Taylor TD, Litt M, Kramer P, et al. (1996) Homozygosity mapping of ) Hallervorden-Spatz syndrome to chromosome 20p12.3-p13. Nature Genetics 14:479-481
- Shan J, Wen B, Zhu J, Lin P, Zheng J, Yan C.(2012) Novel PANK2 gene mutations in two Chinese siblings with atypical pantothenate kinase-associated neurodegeneration. Neurol Sci 29
- Zhou B, Westaway SK, Levinson B, Johnson MA, Gitschier J, Hayflick SJ.A novel pantothenate kinase gene (PANK2) is defective in Hallervorden-Spatz syndrome. Nat Genet. 2001 Aug;28(4):345-9.
- Siudeja K, Grzeschik NA, Rana A, de Jong J, Sibon OCM (2012) Cofilin/Twinstar Phosphorylation Levels Increase in Response to Impaired Coenzyme A Metabolism. PLoS ONE 7(8): e43145
- Fermin-Delgado R, Roa-Sanchez P, Speckter H, Perez-Then E, Rivera-Mejia D, Foerster B, Stoeter P (2012). Involvement of Globus Pallidus and Midbrain Nuclei in Pantothenate Kinase-Associated Neurodegeneration : Measurement of T2 and T2* Time. Clinical Neuroradiology.
- Delgado RF, Sanchez PR, Speckter H, Then EP, Jimenez R, Oviedo J, Dellani PR, Foerster B, Stoeter P. J (2012) Missense PANK2 mutation without “eye of the tiger” sign: MR findings in a large group of patients with pantothenate kinase-associated neurodegeneration (PKAN). Magn Reson Imaging. 35 (4):788-94.
- Hortnagel, K ; Prokisch, H ; Meitinger,T. An isoform of hPANK2, deficient in pantothenate kinase-associated neurodegeneration, localizes to mitochondria. Human Molecular Genetics, 12(3), 321-327
- Kuo, YM, Hayflick, SJ, Gitschier, J (2007). Deprivation of pantothenic acid elicits a movement disorder and azoospermia in a mouse model of pantothenate kinase-associated neurodegeneration. Journal of inherited metabolic disease 30:3 310-31.
- Rana, A., Seinen, E., Siudeja, K., Muntendam, R., Srinivasan, B., van, d. W., et al. (2010). Pantethine rescues a drosophila model for pantothenate kinase–associated neurodegeneration. Proceedings of the National Academy of Sciences of the United States of America, 107(15), 6988-6993.
- Miller-Fleming, L., Giorgini, F. and Outeiro, T. F. (2008), Yeast as a model for studying human neurodegenerative disorders. Biotechnology Journal, 3: 325–338. doi: 10.1002/biot.200700217
- Olzhausen, J., Schubbe, S., Schuller, H. (2009). Genetic analysis of coenzyme A biosynthesis in the yeast Saccharomyces cerevisiae: identification of a conditional mutation in the pantothenate kinase gene CAB1. CURRENT GENETICS, (55), 2: 163-173.
- Garcia M, Leonardi R, Zhang Y-M, Rehg JE, Jackowski S (2012) Germline Deletion of Pantothenate Kinases 1 and 2 Reveals the Key Roles for CoA in Postnatal Metabolism. PLoS ONE 7(7): e40871. doi:10.1371/journal.pone.0040871
- Outeiro, T. F., & Lindquist, S. (2003). Yeast cells provide insight into alpha-synuclein biology and pathobiology. Science, 302(5651), 1772-1775.
- Yagi, T., Kosakai, A., Ito, D., Okada, Y., Akamatsu, W., Nihei, Y., et al. (2012). Establishment of induced pluripotent stem cells from centenarians for neurodegenerative disease research. PLoS ONE, 7(7), 1-7.
- Ananiev G, Williams EC, Li H, Chang Q (2011) Isogenic Pairs of Wild Type and Mutant Induced Pluripotent Stem Cell (iPSC) Lines from Rett Syndrome Patients as In Vitro Disease Model. PLoS ONE 6(9): e25255.
- Batista, L. F. Z., Pech, M. F., Zhong, F. L., Nguyen, H. N., Xie, K. T., Zaug, A. J., et al. (2011). Telomere shortening and loss of self-renewal in dyskeratosis congenita induced pluripotent stem cells. Nature, 474(7351), 399-402.
- Unternaehrer, J. J., Daley, G. Q. (2011). Induced pluripotent stem cells for modeling human diseases. Phil. Trans. R. Soc. B, 366, 2274-2285: doi:10.1098/rstb.2011.0017.
- Wu, S. M., Hochedlinger, K. (2011). Harnessing the potential of induced pluripotent stem cells for regenerative medicine. Nature Cell Biology, 13(5), 497-505.
- Jeong, B. K., Greber, B., Araúzo-Bravo, M. J., Meyer, J., Park, K. I., Zaehres, H., et al. (2009). Direct reprogramming of human neural stem cells by OCT4. Nature, 461(7264), 649-653.
- Sharma, M. C., Aggarwal, N., Bihari, M., Goyal, V., Gaikwed, S., Vaishya, S., et al. (2005). Hallervorden spatz disease: MR and pathological findings of a rare case. Neurology India, 53(1), 102-104.
- Pluropotent Stem Cell Facility. (2011). Cincinnati Children’s Hospital Medical Center. https://research.cchmc.org/stemcell/iPSC.