Detecting the danger: How do moths and butterflies manage to escape their predators?
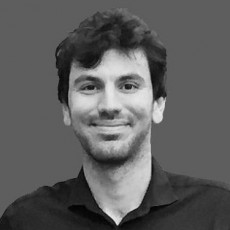
Joshua Spreng
Department of Biology
Lake Forest College
Lake Forest, IL 60045
Introduction
Hearing organs are essential sensory systems for intraspecific communication as well as for detection and avoidance of predators. Using technological as well as molecular methods, studies provide knowledge and a better understanding of the structure as well as of the evolutionary development process of acoustic sensory organs in animals, especially in insects. The ability to detect acoustic signals generated by prey, predator, competitors (rivals) and applicable mates is crucial for survival and reproduction (Hoy, 1996; Yager, 1999). The bat – moth model is a significant representative of coevolution and leads to a better understanding of prey – predator relationships and how organisms respond and adapt – behaviorally as well as morphologically – to their environment and increased predator pressure. Some nocturnal flying insects developed ears that are sensitive to ultrasound as a response to the echolocation predation strategies of various bats and can therefore be seen as a product of evolutionary adaptation (Windmill, 2006). Studies have also shown that nocturnal insects not only perform escape maneuvers after the detection of predators but also actively send back signals in order to startle and irritate the predators (Fournier, 2013; Miller, 2001; Corcoran, 2011; Nakano, 2015). Furthermore, studies have revealed that bats themselves have responded to the evolved detection and interference strategies of moth as well by changing the frequency and / or amplitude of signals during echolocation and by listening to the sound produced during the prey’s escape movements (Miller, 2001; Conner, 2011). Researching such species interactions not only increases the knowledge of how different organisms influence each other, but also informs us about evolution on a larger scale.
Anatomy of the acoustic sensory organ in insects: The tympanate ear
The sensory part of the auditory system of animals consists of the tympanate ear, which acts as a pressure detector and is found in a variety of organisms, from invertebrates to vertebrates (Yager, 1999). However, compared to vertebrates, the auditory sensory organs of insects are present in a wide variety and are not located near the center of the nervous system (cranial), but are found on various locations on their bodies (Hoy, 1996; Kamikouchi, 2013). The locations are different among various insect species and may even vary within a particular species: In crickets, for example, the tympanate ears are located on the forelegs (Geurten, 2013; Poltnick, 2012), in grasshoppers, on the first abdominal segment (Plotnick, 2012), in butterflies, near the wings (Lucas, 2014), in moths, on the first abdominal segment, at the base of the forewings or at the anterior or posterior region of the second abdominal segment (Pfuhl, 2015). The tympanate ears consist of three anatomical parts: the tympanum, the tracheal sac, and the tympanal organ. The tympanum is a thin skin layer that will vibrate if there is any change in pressure detected. The tracheal sac is an air filled sac inside of the tympanum, which increases the frequency of the vibration, but is not responsible for tuning or directionality (Yager, 1999). The tympanum is responsible for converting the changes in pressure to motions of the membrane. The tympanal organ is in charge of transcribing or changing the produced mechanical signal into a neural signal (Yager, 1999). Scientists have determined two main causes that are responsible for the development and evolution of tympanate ears. The first is intra species communication through sound recognition and localization. The second is the detection of predators, particularly bats, by being sensitive to ultrasound (Hoy & Robert, 1996; Stumpner & Helversen, 2001; Senter, 2008).
Detection of predators and escape maneuvers
Butterflies and moths are related insects in terms of evolution. They are both members of the order Lepidoptera . By generating a molecular dataset, using a phylogenomic analysis, scientists were able to establish a close relationship between moths and butterflies, which contradicted the historical placement of butterflies - data of nucleotides and amino acid yielding nearly identical and transcriptome-based trees are well supported (Kawahara, 2014). Moths and butterflies do not only share similar characteristics such as wings that are covered with scales, but both own hearing organs that are specially adapted to detect the sounds that are generated by their predators (Fournier, 2013). Since most of the butterflies are day active, their ears are sensitive to day active predators. Nymphalid butterflies (Morpho peleides) and crepuscular owl butterflies (Caligo Eurilochus) for example have a frequency sensitivity of 1kHz and 1 - 4 kHz, respectively, and are therefore able to detect the approach of eastern phoebe (Sayornis phoebe) and the chickadee (Poecile atricapillus) whose frequency of wing flapping is 18.5 +/- 0.19 kHz and 20.7 +/- 2.5 kHz, respectively (Fournier, 2013; Lucas, 2014). Playbacks of recorded flight sounds of an attacking bird lead in a signal transportation across auditory nerve cells of both noctuid moths and nymphalid butterflies, resulting in escaping maneuvers of the organisms (Fournier, 2013).
As a response to the increasing predation pressure by insectivorous bats, nocturnal insects evolved sensitivity to the ultrasonic echolocation calls of bats (Nakano, 2015). Most moths have evolved sensitivity to ultrasonic sound between 20-60 kHz at its best and nocturnal butterflies of Hedyloidea have shown sensitivity between 40 kHz and 80 kHz (Nakano, 2015: Lucas, 2014). Other insects such as crickets, katydids, beetles, and lacewings are able to detect the sonar radiation of bats and perform various behavior forms in response to it (Conner, 2011). Since species of moths differ greatly in size, there is a correlation between body size and sensitivity, i.e. best heard frequency: The ears of larger sized moths are tuned to be more sensitive (Nakano, 2015; Miller, 2001). Although large moths reflect an intense echo due to their body size and are therefore detectable by bats on a distance of up to 10 m, those moths are capable of detecting bats over a distance of 100 m due to their adapted sensitivity (Miller, 2001). Exposed to imitating sounds of bat calls, moths have shown a variety of escaping movements consisting of turning and flying away, zigzag and looped-shaped flights, and abrupt and powerful dives or passive falls (Miller 2001; Conner 2012).
Strategies to avoid predators’ attacks: Consternation, warning and masking
Besides the escape movements after detecting predators, studies have shown that nocturnal insects such as moths have other strategies that prevent them from being eaten and therefore contribute to their survival. Some moth species developed sound producing organs, tymbals, which are not only used in order to communicate with other individuals in the species (i.e. through courtship calls in order to reproduce), but are also being used to agitate and / or irritate attacking bats (Nakano, 2015). In order to confuse bats, male as well as female moths respond to the echolocation calls of bats by emitting a single or a series of click calls in the ultrasonic range (Nakano, 2015). Those click calls have three hypothesized main purposes: 1.) Causing the bats to interpret moth clicks as echoes (known as phantom echo hypothesis), 2.) Decreasing the bats’ precision during the distance evaluation process (known as ranging interference hypothesis), and 3.) Fully masking the echo of the moths, making them temporarily “invisible” for bats (known as the masking hypothesis) (Corcoran, 2011). Analysis of the bats response in terms of echolocation and flight movement support the ranging hypothesis (Corcoran, 2011). Moths, such as tiger moths, not only seem to borrow the bats’ strategy of distance and location strategy by evaluating the repetition rate of the bat calls to the clicking signals, but also interfere and interrupt the bat’s precise echolocation technique by sending long series of high density clicks that overlap with the bats’ own echo (Nakano, 2015). Furthermore, studies have shown that some moths also use sounds as acoustic warnings. Arctiid moths, for example, are toxic and use single click calls in order to extend their supplemental visual warning, which consist of bright colors (Nakano, 2015). The fact that moths do not represent the main part of the diet of bats may explain the effectiveness of the escape and defense strategies of moths (Miller, 2001).
Countering strategies and adaptations: Predators respond
Scientists stated possible explanations of adaptations and counter strategies which would allow bats to avoid the defense mechanisms of moths. One possible counter tactic is changing the frequency during echolocation, i.e. moving the sonar frequency out of the range of the sensitivity of tympanate ears of insects by using higher or lower frequencies (Miller, 2001; Conner 2011). There is evidence that some bats are emitting echolocation signals with an increased frequency that have the effect of overcoming insect detection abilities (Miller, 2001; Goerlitz,
2010; Conner 2011). However, since an increase in frequency and / or amplitude also results in an increase of the resolution of the echolocation process, it is not yet clear whether this behavior represents an adaptation as a response to the moth hearing or a general improvement of the sonar technique (Miller, 2001; Goerlitz, 2010; Conner 2011). Studies have shown that aerial-hawking bats, for example, emit echolocation signals of a high – amplitude nature in order to expand their detection range, which may result in an increase of the bats’ ability to detect and catch their prey (Goerlitz, 2010). Through bat flight-path and moth neurophysiology analyses, scientists were able to show that bats of Barbastella barbastellus perform a counter strategy, known as stealth echolocation, by emitting signals with amplitudes that are 10 to 100 times lower than the ones of aerial-hawking bats and which are undetectable by moths, resulting in significant capturing success (Goerlitz, 2010; Conner, 2011). This technique gives Barbastella an advantage over other ariel-hawking bats, but it also comes with a cost: it reduces the detection distance (Goerlitz, 2010). A second strategy could be the changing of the intensity of the signals during echolocation: Since intensity of sound is proportional to the pressure of the sound, bats will be less detectable to the tympanate ear of the insect (Miller, 2001). Listening to the sound produced by the prey while moving around or by moving their wings can be seen as a third strategy (Miller, 2001). However, this strategy has its limits and does not work for organisms that stay motionless: Sedentary moths, for example, have been found to stay motionless or freeze upon hearing of echolocation signals (Miller, 2001). To decide if the behavior of bats turning off echolocation can be seen as a counter strategy is difficult. Miller (2001), for example, states that it is possible that bats stop the echolocation process since they do not require further updates on the estimated position of the resting prey. Furthermore, scientists were able to show that some predators made modifications on their wings, in order to be acoustically undetectable by prey. Barn owls (Tyto alba), for example, feed on moths and have developed specific feather arrangements that significantly reduce noise that is produced while hunting (Sarradj, 2011; Fournier 2013).
Conclusion and Future Studies
The research performed by various scientists show that the ability of insects, such as moths and butterflies, to detect acoustic signals that are generated by other organisms is not only crucial in terms of intra-species communication and reproduction but also in terms of surviving. The development of hearing systems and their adaptations, the evolvement of strategies to prevent a predator’s attack, and the counter strategies of predators are all evolutionary processes. The moth – bat relationship is a prime example of this. In order to detect and escape predators, moths and butterflies have evolved and adapted hearing organs and are capable of performing a variety of escape maneuvers. Furthermore, some moths developed strategies to either confuse or warn bats or to make themselves temporally “invisible”. Bats, on the other hand, have evolved behavioral counter strategies to possibly avoid the defense mechanisms of moths. Some of them can increase the bats’ ability to detect and catch moths while others are only effective to a certain degree (i.e. some moths stay motionless if they detect a predator and are therefore difficult to be distinguished by the predator itself). Future research is required to decide whether the behavior of turning off echolocation can be seen as a counter strategy and if predators have adapted morphologically, i.e. by modifying their feathers in terms of sound reduction and their attacking behaviors (Miller, 2001; Sarradj, 2011; Fournier, 2013).
The scientists’ findings not only contribute to a better understanding of how ears in insects, such as moths and butterflies, evolved and are used to detect predators in order to maintain survival, but also of how and to what degree organisms put evolutionary pressure on each other. This may lead to an increase in knowledge about predator/prey relationships, how organisms adapt and respond to their environment (including other organisms) in order to survive, and why organisms behave the way they do. On an even larger scale, these results may provide a better understanding of the concept of evolution.
Eukaryon, Vol. 12, March 2016, Lake Forest College
References
Conner, W. E., & Corcoran, A. J. (2012). Sound strategies: the 65-million-year-old battle between bats and insects. Annual review of entomology, 57, 21-39.
Corcoran, A. J., Barber, J. R., Hristov, N. I., & Conner, W. E. (2011). How do tiger moths jam bat sonar? The Journal of experimental biology, 214(14), 2416-2425.
Fournier, J. P., Dawson, J. W., Mikhail, A., & Yack, J. E. (2013). If a bird flies in the forest, does an insect hear it? Biology letters, 9(5), 20130319.
Geurten, B., Spalthoff, C., & Göpfert, M. C. (2013). Insect hearing: Active amplification in tympanal ears. Current Biology, 23(21), R950-R952.
Goerlitz, H. R., ter Hofstede, H. M., Zeale, M. R., Jones, G., & Holderied, M. W. (2010). An aerial-hawking bat uses stealth echolocation to counter moth hearing. Current Biology, 20(17), 1568-1572
Hoy, R. R., & Robert, D. (1996). Tympanal hearing in insects. Annual review of entomology, 41(1), 433-450.
Kamikouchi, A. (2013). Auditory neuroscience in fruit flies. Neuroscience research, 76(3), 113-118.
Kawahara, A. Y., & Breinholt, J. W. (2014). Phylogenomics provides strong evidence for relationships of butterflies and moths. Proceedings of the Royal Society of London B: Biological Sciences, 281(1788), 20140970.
Lucas, K. M., Mongrain, J. K., Windmill, J. F., Robert, D., & Yack, J. E. (2014). Hearing in the crepuscular owl butterfly (Caligo eurilochus, Nymphalidae). Journal of Comparative Physiology A, 200(10), 891-898.
Lucas, K. M., Windmill, J. F., Robert, D., & Yack, J. E. (2009). Auditory mechanics and sensitivity in the tropical butterfly Morpho peleides (Papilionoidea, Nymphalidae). Journal of Experimental Biology, 212(21), 3533-3541.
Miller, L. A., & Surlykke, A. (2001). How some insects detect and avoid being eaten by bats: tactics and countertactics of prey and predator evolutionarily speaking, insects have responded to selective pressure from bats with new evasive mechanisms, and these very responses in turn put pressure on bats to “improve” their tactics. Bioscience, 51(7), 570-581.
Nakano, R., Takanashi, T., & Surlykke, A. (2015). Moth hearing and sound communication. Journal of Comparative Physiology A, 201(1), 111-121.
Pfuhl, G., Kalinova, B., Valterova, I., & Berg, B. G. (2015). Simple ears-flexible Information processing in the moth auditory pathway. Current Zoology, 61, 292-302.
Plotnick, R. E., & Smith, D. M. (2012). Exceptionally preserved fossil insect ears from the Eocene Green River Formation of Colorado. Journal of Paleontology, 86(1), 19-24.
Sarradj, E., Fritzsche, C., & Geyer, T. (2011). Silent owl flight: bird flyover noise measurements. AIAA journal, 49(4), 769-779.
Senter, P. (2008). Voices of the past: a review of Paleozoic and Mesozoic animal sounds: review. Historical Biology, 20(4), 255-287.
Stumpner, A., & Von Helversen, D. (2001). Evolution and function of auditory systems in insects. Naturwissenschaften, 88(4), 159-170.
Windmill, J. F. C., Jackson, J. C., Tuck, E. J., & Robert, D. (2006). Keeping up with bats: dynamic auditory tuning in a moth. Current biology, 16(24), 2418-2423.
Yager, D. D. (1999). Structure, development, and evolution of insect auditory systems. Microscopy research and technique, 47(6), 380-400.