Changes in Behavior following Extinction: the Effects on Predator Species versus Prey Species
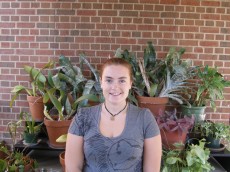
Jennifer Sojka
Department of Biology
Lake Forest College
Lake Forest, Illinois 60045
Download PDF
Introduction
Single species extinctions can have rippling effects on the ecosystem as a whole. The effects of the extinction of a keystone species, for example, can ripple through the ecosystem by releasing conspecifics from competition or disrupting ecosystem functions, while the disappearance of a mutualist can spell the demise for the species who relied on them (Koh et al., 2004; Schulze & Mooney, 1993) . Yet, the effects are often considerably less dramatic. In particular, subtle changes in behavior of conspecifics often follow the extinction of a species. These changes may be especially widespread in species that interact directly, particularly between predator and prey species. Because of the differences in the nature of their relationships, however, the behavioral responses of prey species following the extinction of a predator are fundamentally different than the behavioral changes incurred when predators lose a prey species. Regardless of the direction, small changes in behavior may result in large overall effects that can have significant influences on conservation and ecosystem function.
Responses of Prey
Prey species must be able to recognize their predators. Animals that can do so via sensory input and respond accordingly are more likely to survive and pass on their genetic material to the next generation. However, when pressures are relaxed following the extinction or extirpation of predators, the ability to recognize predators no longer carries a selective advantage. As a result, recognition behavior starts to decay and can eventually disappear altogether (Orrock, 2010).
The time involved in the disappearance of recognition varies according to how costly it is to maintain the recognition mechanism (Blumstein, 2006). Unused senses that are metabolically costly should be quickly selected against. For instance, if a squirrel uses a large amount of its total energy to maintain sharp hearing so that it can quickly detect approaching snakes, then the extinction of the predator should lead to a fast decline in aural capabilities. In this case, the now unused energy can be diverted to areas that could increase survival or reproductive success.
Decay in recognition is also confounded by other advantages that the same recognition may lend that are unrelated to predator avoidance. In the previous example, the same squirrel may use auditory cues to recognize members of its social group. As a result, hearing may not deteriorate as fast as predicted following the extinction of the snake.
Three types of predator recognition are primarily studied in behavioral ecology: visual, auditory and olfactory. Vision, to begin with, is of primary importance in many divisions of the animal kingdom and plays a significant role in predator recognition (Bearder et al., 2006; Rowland, 1999). In fact, the diversity of the eye may have originally radiated in response to an increase in predation pressure (Fernald, 2000). Because of its significant role in detection, the loss of visual recognition of predators has been studied in several organisms. Several studies have used taxidermic mounts, cutouts, and exposure to live animals to study the loss of visual recognition of predators (Blumstein, 2002; Blumstein et al., 2009; Li et al., 2011; Peer et al., 2011; Stankowich & Coss, 2007). These studies have largely found that visual recognition is maintained (Stankowich & Coss, 2007). The amount of time that the predators were absent ranged from 70 years to 2.8 million years (Blumstein et al., 2009; Peer et al., 2011). Thus, the persistence of visual recognition of predators has been demonstrated to occur over a wide range of timescales. Overall, this evidence suggests that visual recognition does not decay despite relaxed predation pressure.
Olfactory recognition is also an important factor in recognizing potential predators. Studies suggest that prey can recognize the sulfur content of predator urine and feces and respond accordingly (Nolte et al., 1994). Notably, smell is an indirect cue. That is, recognizing a scent can only tell a prey animal that a predator had, at one point, been present at the site, not if it is currently there (Fletcher & Boonstra, 2006). This could be a confounding factor in experiments that test olfactory recognition because prey species may detect the scent but choose not to respond.
The rate or degree at which olfactory recognition is lost following extinctions of predators does not seem to have a uniform pattern. For instance, experiments have demonstrated that some animals retained their ability to discern predators via smell, while others have lost it (Berger et al., 2001; Blumstein, 2002; Blumstein, Barrow, & Luterra, 2008; Orrock, 2010). Additionally, the length of time that prey retain olfactory recognition varies greatly. For instance, moose lost their ability to recognize both bear and wolf feces after less than five generations (Berger et al., 2001; Gaillard 2007). In contrast, yellow-bellied marmots retained the recognition of predators that had been extinct for more than eight generations (Blumstein et al., 2008; Schwartz et al., 1998). This evidence emphasizes that more research is needed in this area to determine what may cause the relatively quick decay of olfactory recognition in some species while it persists in others.
Finally, prey species may lose the ability to recognize auditory signals from their extinct or extirpated predators. Again, there are disparities between how long and to what extent animals lose the ability to discern predator vocalizations from background noise. Some studies have shown that the decay of auditory recognition is possible (Li et al., 2011; Yorzinski & Ziegler, 2007). However, despite 130 years of complete isolation from predators, tammar wallabies continue to alter foraging and vigilance behaviors in response to predator vocalization (Blumstein, 2002).
There are several hypotheses to explain why some prey species retain antipredator behavior while others do not. Although these theories rarely address the maintenance of recognition directly, most can be extended to include it. To begin with, the “ghost of predation past” hypothesis suggests that antipredator behaviors remain simply because they are not actively selected against in the population (Peckarsky & Penton, 1988). As discussed earlier, the ability to recognize predators may be relatively inexpensive to maintain because it is incorporated in a suite of behaviors that are beneficial in other situations.
An alternative to the “ghost of predators past” hypothesis has emerged relatively recently in the literature. This Multipredator Hypothesis, as the name suggests, predicts that as long as animals remain vulnerable to predation of any kind, they will retain all antipredator behavior and recognition, even for extinct predators. This theory is based on the assumption that the genetic coding for complex traits that are pervasive through evolutionary time in multiple phyla (such as antipredator responses) should lie close to each other on the genome. Because linked traits do not sort independently, the maintenance of one type of antipredator response would “protect” others (Blumstein, 2006).
These hypotheses, however, assume that recognition of predators is innate. However, fear of predators can be conditioned in the lab (Cook & Mineka, 1988). Furthermore, naïve animals, such as those that have been isolated on islands, have been shown to significantly change their behavior after limited experiences with reintroduced predators (Berger et al., 2001). While it is probable that many aspects of predator recognition are inherited, these experiments demonstrate that it can be at least partially learned. The degree to which recognition is learned or innate may additionally explain the disparity in lengths of time that it is maintained.
In addition to losing the ability to recognize predators, prey species also alter foraging patterns in response to predator extinctions. This response is known as a behaviorally mediated trophic cascade (BMTC) (Schmitz, Beckerman, & O’Brien, 1997). A BMTC occurs when the loss of one species sets off a ripple effect that alters the behavior of other species, eventually causing changes in the system as a whole. For example, elk in the western United States have been documented to abandon risk sensitive foraging patterns in the absence of wolves (Ripple & Beschta, 2006). This change in behavior has even been shown to alter densities of cottonwood, willow and aspen growth (Ripple & Beschta, 2003; Ripple & Beschta, 2006; Kauffman et al., 2010). By changing the amount of canopy cover, elk can effectively change forests into meadows, thus fundamentally altering the structure of the community.
Responses of Predators
Predators have also been observed to display a number of behaviors following the extinction of prey species. To begin with, although predators may also use visual, olfactory and auditory cues to find prey, there is no evidence to suggest that predators lose their ability to recognize extinct or extirpated species of prey. This may be because many predators are generalists and prey on several species. When one species goes extinct, they still have to capture others to persist. Because they may respond to similar cues from multiple species, such as general size or shape, predators may not ever lose visual recognition of an extinct prey species.
Prey switching, however, is a behavior that can be predicted. Because most predators are not specialists, the effects of prey switching are often unnoticed or misinterpreted. For example, the significant loss in seals, sea lions, and otters of the North Pacific was initially blamed on increased pollution. However, it was later revealed that the decline actually was the result of prey switching in the killer whale, whose natural prey, the great whales, were being hunted to near extinction (Springer et al., 2003).
In some cases, however, predators may not have a prey to shift to. This is the case for the Iberian Lynx and Spanish Imperial Eagle. Their main prey, the European rabbit, is continuing to decline to due to widespread disease (Ferrer & Negro, 2004). Because the lynx and the eagle are both specialists, and prey only on the rabbit, the three may be headed down the path toward extinction together.
Predators have also been documented changing other aspects of their foraging behavior. For instance, they can aggregate around declining prey populations. This has been observed in the Iberian Lynx and the Spanish imperial eagle who have followed decreasing populations of rabbits in Western Europe (Delibes-Mateos et al., 2007). Interestingly, this can have large-scale biogeographic and political repercussions, as large predators increase their density in areas where they may not be wanted. Furthermore, with a decrease in prey species, predators may even change their searching strategies. For instance, lynx increase their foraging rapidly when hare density drops below one hare per hectare effort by spending more time hunting and traveling each day (Ward & Krebs, 1985).
Interestingly, the literature on the response of predators to the extinction of prey species is considerably sparser than that of the reaction of prey to predator extinction. For example, despite being one of the most common birds in North America, there are no recorded predator responses to the extinction of the passenger pigeon (Sekercioglu, 2006). Additionally, the heath hen was an abundant source of prey in colonial America. However, there is no documented case of a single effect on predator behavior in its absence.
There may be several reasons that explain this imbalance in research. To begin with, predators are much more difficult to study. They tend to be more rare and more spread out. As a result, they may need to be monitored using expensive radio collars or difficult tracking techniques. Finally, predators may also be considerably more dangerous than prey species. Consequently, behavioral studies that involve directly observing or even approaching the animal may be ruled out due to concerns for the safety of researchers.
Conclusions and Implications
First and foremost, this research points to the fact that extinctions, especially those caused relatively quickly or recently by humans, can have significant effects on the ecosystem beyond the loss of just one species. The loss of one species may cause behavioral changes in its conspecifics that lead to further extinctions. This extinction would in turn create the same adverse effects and lead to a cycle that may force many species down the path to extinction. Moreover, the loss of a single predator or prey species can significantly alter the structure of ecosystems. This could easily lead to the breakdown of whole systems and the loss of valuable ecosystem services.
Finally, this research adds an additional complication to conservation efforts. In particular, it is important to scrutinize reintroduction projects with regards to X, Y, and Z. Although reintroduction of prey species into a previously occupied habitat may have few effects on its former predators who have ecologically moved on, the restoration of predators to historic ranges may upset a newly established balance. This is especially pertinent if the potential prey species of the reintroduced predators are vulnerable to extinction.
Overall, predators and prey experience different behavioral changes following extinctions. While prey species most notably lose the ability to recognize predators through hearing, sight and small, the decay of extinct prey recognition by predators has not been adequately documented. Furthermore, the behavioral changes in prey species can take a considerable amount of time. On the other hand, predators often have to take quick, drastic measures, such as prey switching or aggregating, in order to survive the extinction of one of their prey species. It is also worth mentioning that both predator and prey species experience alterations in foraging behavior following extinctions.
However, these too are fundamentally different processes. Prey species alter their behavior in response to a loss of fear of predators. In contrast, predators again change their foraging in order to survive. Because of these differences, it may be much more important for predators to have considerable plasticity in their foraging behavior, while not as crucial for prey. However, more research is needed in this area of behavioral ecology in order to present a clear picture of what happens to the behaviors of prey species and predators following extinctions.
References
Bearder, S. K., Nekaris, K. A. I., & Curtis, D. J. (2006). A re-evaluation of the role of vision in the activity and communication of nocturnal primates. Folia Primatologica, 77, 50–71.
Berger, J., Swenson, J. E., & Persson, I. L. (2001). Recolonizing carnivores and naïve prey: Conservation lessons from Pleistocene extinctions. Science, 291, 1036–1039.
Blumstein, D. T. (2002). Moving to suburbia: Ontogenetic and evolutionary consequences of life on predator-free islands. Journal of Biogeography, 29, 685–692.
Blumstein, D. T. (2006). The multipredator hypothesis and the evolutionary persistence of antipredator behavior. Ethology, 112, 209–217.
Blumstein, D. T., Barrow, L., & Luterra, M. (2008). Olfactory predator discrimination in yellow-bellied marmots. Ethology, 114, 1135–1143.
Blumstein, D. T., Ferando, E., & Stankowich, T. (2009). A test of the multipredator hypothesis: Yellow-bellied marmots respond fearfully to the sight of novel and extinct predators. Animal Behaviour, 78, 873–878.
Cook, M., & Mineka, S. (1988). Observational conditioning of fear to fear-relevant versus fear-irrelevant stimuli in rhesus monkeys. Journal of Abnormal Psychology, 98, 448–459.
Coss, R. G. (1991). Context and animal behavior III: The relationship between early development and evolutionary persistence of ground squirrel antisnake behavior. Ecological Psychology, 3, 277–315.
Delibes-Mateos, M., Redpath, S. M., Angulo, E., Ferreras, P., & Villafuerte, R.. (2007). Rabbits as a keystone species in southern Europe. Biological Conservation, 137, 149–156.
Fernald, R. D. (2000). Evolution of eyes. Current Opinion in Neurobiology, 10, 444–50.
Ferrer, M., & Negro, J. J. (2004). The near extinction of two large European predators: Super specialists pay a price. Conservation Biology,18, 344–349.
Fletcher, Q. E., & Boonstra, R. (2006). Do captive male meadow voles experience acute stress in response to weasel odour? Canadian Journal of Zoology, 588, 583–588.
Gaillard, J. (2007). Are moose only a large deer?: Some life history considerations. Alces, 43, 1–11.
Kauffman, M. J., Brodie, J. F., & Jules, E. S. (2010). Are wolves saving Yellowstone’s aspen? A landscape-level test of a behaviorally mediated trophic cascade. Ecology, 91, 2742–55.
Koh, L. P., Dunn, R. R., Sodhi, N. S., Colwell, R. K., Proctor, H. C., & Smith, V. S. (2004). Species coextinctions and the biodiversity crisis. Science, 305, 1632–1634.
Li, C., Yang, X., Ding, Y., Zhang, L., Fang, H., Tang, S., & Jiang, Z. (2011). Do Père David’s deer lose memories of their ancestral predators? PLoS ONE, 6, e23623.
Nolte, D., Mason, J., Epple, G., Aronov, E., & Campbell, D. L. (1994). Why are predator urines aversive to prey? Journal of Chemical Ecology, 20, 1505–1516.
Orrock, J. L. (2010). When the ghost of predation has passed: Do rodents from islands with and without fox predators exhibit aversion to fox cues? Ethology, 116, 338–345.
Peckarsky, B. L., & Penton, M. A. (1988). Why do ephemerella nymphs scorpion posture: A “ghost of predation past”? Oikos, 53, 185.
Peer, B. D., Kuehn, M. J., Rothstein, S. I., & Fleischer, R. C. (2011). Persistence of host defence behaviour in the absence of avian brood parasitism. Biology Letters, 7, 670–673.
Ripple, W. J., & Beschta, R. L. (2003). Wolf reintroduction, predation risk, and cottonwood recovery in Yellowstone National Park. Forest Ecology and Management, 184, 299–313.
Ripple, W. J., & Beschta, R. L. (2006). Linking wolves to willows via risk sensitive foraging by ungulates in the northern Yellowstone ecosystem. Forest Ecology and Management, 230, 96–106.
Rowland, W. J. (1999). Studying visual cues in fish A review of ethological techniques. Environmental Biology of Fishes, 56, 285–305.
Schmitz, O., Beckerman, A., & O’Brien, K. (1997). Behaviorally mediated trophic cascades: Effects of predation on food web interactions. Ecology, 78, 1388–1399.
Schulze, E., & Mooney, H. A. (Eds.). (1993). Biodiversity and Ecosystem Function. (1st ed.). Berlin, Germany: Springer-Verlag.
Schwartz, O. A., Armitage, K. B., & Van Vuren, D. (1998). A 32-year demography of yellow-bellied marmots (marmota faviventris). The Zoological Society of London, 246, 337–346.
Sekercioglu, C. H. (2006). Increasing awareness of avian ecological function. Trends in Ecology & Evolution, 21, 464–71.
Springer, A. M., Estes, J. A., van Vliet, G. B., Williams, T. M., Doak, D. F., Danner, E. M.Pfister, B. (2003). Sequential megafaunal collapse in the North Pacific Ocean: An ongoing legacy of industrial whaling? Proceedings of the National Academy of Sciences of the United States of America, 100, 12223–12228.
Stankowich, T., & Coss, R. G. (2007). The re-emergence of felid camouflage with the decay of predator recognition in deer under relaxed selection. Proceedings of the Royal Society of London, B 274, 175–82.
Ward, R. M. P., & Krebs, C. (1985). Behavioral responses of lynx to declining snowshoe hare abundance. Canada Journal of Zoology, 63, 2817-2824.
Yorzinski, J. L., & Ziegler, T. (2007). Do naïve primates recognize the vocalizations of felid predators? Ethology, 113, 1219–1227.