A New Protein Target: Finding Optineurin’s Role in Amyotrophic Lateral Sclerosis (ALS)
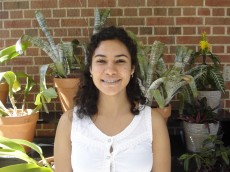
Daysi Vargas, Sydni Cole, Shabana Yusufishaq
Department of Biology
Lake Forest College
Lake Forest, Illinois 60045
Download PDF
Abstract
Amyotrophic Lateral Sclerosis (ALS) is a progressive neurodegenerative disorder that leads to motor neuron death in the brain and spinal cord. A number of genes have been associated with the cause of familial ALS (FALS), including SOD1 and TDP-43. Recently, OPTN, a gene linked to posterior open angle glaucoma, was found to be associated with FALS (19). In the E478G missense mutation of OPTN, its protein, optineurin, interacts with SOD1 and TDP-43 inclusions, as well as the transcription factor NF-kB. Although this suggests that mutations on the OPTN gene result in ALS pathogenesis, the function of OPTN and its implication on other genes’ regulation is unknown. In this proposal, we hypothesize that OPTNE478G acts as a gain of function mutation and interacts with motor neuron genes, TDP-43, NF-kB, and SOD1; we will validate our hypothesis using a mouse model. We will first create a transgenic mouse model of OPTNE478G to study if said mutation leads to ALS pathology. The transgenic mice will be studied in relation to control mice and OPTN gene knockout mice to track disease progression by studying behavior, pathology, and subcellular activity. Given that little is known about OPTN’s possible effect on regulation of motor neuron genes, we will also investigate which motor neuron genes, in the presence of OPTNE478G, are overexpressed and underexpressed by using a microarray assay. Third, we will determine if, in fact, OPTN interacts with SOD1 and TDP-43 to promote motor neuron degeneration through the process of immunohistochemistry analysis. Determining the extent to which OPTN plays a role in ALS will provide great insight into ALS pathogenesis.
Background
300,000 individuals in the United States and 4 in every 100,000 people worldwide are affected by Amyotrophic Lateral Sclerosis (ALS), most commonly known as “Lou Gehrig’s Disease.” ALS is characterized by gradual motor neuron death in the brain and spinal cord, resulting in muscular weakness and respiratory failure. The onset of the disease is between ages 40-70, leading to death 2 to 5 years thereafter. Interestingly, ALS seems to affect more males than females and has a particular affinity for Caucasians (12).
ALS like other neurodegenerative diseases is often classified into two categories, familial, which affects 10-15% of patients, and sporadic, which affects 85-90% of patients. Genetic mutations of FALS include: ANG encoding angiogenin, TARDP encoding TAR, FUS/TLS, TDP-43, and SOD1 (19). Of these mutations, the autosomal dominant SOD1 mutations account for 20% of FALS cases (28). SOD1 has been extensively studied and has contributed greatly to the knowledge of FALS and SALS thus far (7) because the pathology found in FALS is also found in SALS. SOD1 mutations lead to mitochondrial stress, dysfunction, and ultimately, cell death (15). Recently, it was discovered that mutations in OPTN might also contribute to cases of FALS (19).
The OPTN gene is most formerly associated with primary open angle glaucoma (POAG) and normal tension glaucoma (NTG), but this is not the case with neurodegenerative diseases (9). The gene is located on chromosome 10p14-p15 (26). It has 16 exons: 3 are non-coding and the other 13 code for a 577-amino acid protein (9) called optineurin (26). The specific mutation of OPTN that is most studied, OPTNE50K, is the one most commonly associated with glaucoma (9). OPTNE50K and OPTNwt have been shown to inhibit the TNFa activation of NF-kB (6) by competing with its homologue, NF-kB essential modulator (NEMO), with which it shares a 53% amino acid similarity (10; 38).
Three OPTN mutations have recently been studied in regards to ALS: an exon 5 deletion, the Q398X nonsense mutation, and the E478G missense mutation linked to FALS cases (19). OPTNE478G and OPTNQ398X disinhibit the activation of NF-kB (19). UnderstandingNF-kB disinhibition by OPTNE478G could be key in further understanding the disease and the development of new treatments. OPTNE478G also presents itself in SOD1 and TDP-43 inclusions, suggesting that said mutation might be a characteristic of many types of FALS (19). A gain of function mutation of OPTN would result in excess optineurin protein, thus allowing the excess protein to accumulate in SOD1 and TDP-43 inclusions, as has been previously seen (19).
Creating an animal model of the OPTNE478G mutation will allow us to better understand the interaction of optineurin with SOD1, TDP-43, and NF-kB. Meanwhile, we will also be able to determine if OPTNE478G results in a gain or loss of function of optineurin. Additionally, motor neuron genes affected by OPTNE478G have yet to be identified. We hypothesize that OPTNE478G is a gain of function mutation, that the mutated OPTN gene influences motor neuron genes and that optineurin interacts with motor related proteins such as SOD1, TDP-43, and NF-kB.
Relevance
Broader Relevance: One in 10,000 individuals acquires FALS (9). Despite only one-fourth of patients acquiring FALS, we can understand a great deal about ALS, including SALS, by studying gene interactions of FALS. 20-30% of FALS patients present with studied genetic mutations (11; 19): what about the other 70-80%? As of yet, there is neither a cure nor a way to slow the progression of the disease once symptoms present themselves. The findings of this proposal will expand our knowledge of OPTN’s relation to ALS pathogenesis, thus leading to potential therapies. Moreover, this study will contribute to the training of future scientists.
Intellectual Merit: In this proposal we will be looking at the function of OPTN and how mutant forms of this protein could affect ALS neurodegeneration. We propose to investigate optineurin’s interaction with TDP-43, SOD1, and NF-kB as well as how gene expression is affected by OPTN mutations, not being limited to ALS. It could also potentially provide us with general knowledge of protein interactions. Further understanding OPTN function and its interactions with other proteins and genes will advance the scientific field of ALS and many other neurodegenerative disorders.
Specific Aims
The long-term goal of this project is to clarify OPTN’s role in ALS pathogenesis and to identify possible treatments for ALS. This study will examine the specific function of OPTN and how OPTN’s interaction with other proteins can have a role in ALS pathogenesis. The main hypothesis of this study is that OPTN’s contribution to ALS is due to a gain of function mutation and is linked to interactions with SOD1, TDP-43, NF-kB, and motor neuron genes.
1. To create an animal model of the OPTN mutation in ALS: Four groups of mice will be created—a non-transgenic control mouse, a control transgenic mouse with human OPTNwt, an OPTNE478G transgenic mouse, and an OPTN knockout mouse. They will then be studied at the behavioral, pathological, and sub-cellular levels to determine if the mouse model works well for the OPTN mutation of ALS and if the mutation is, in fact, a gain of function mutation.
2. To characterize WT and mutant optineurin effects on motor neuron genes: We will examine the effect OPTNwt and mutant OPTNE478G have on motor neuron gene expression by utilizing a microarray assay to examine mRNA extracted from mice brain and spinal cord tissue. In addition, we will study optineurin’s interaction with NF-kB by performing a co-immunoprecipitation analysis coupled with an in-vitro pull-down assay.
3. To determine to what extent optineurin interacts with SOD1 or TDP-43 inclusions: Singly mutated transgenic mice, including SOD1, TDP-43, and OPTNE478G will be used to determine co-localization of SOD1/OPTN and TDP-43/OPTN. The influence of double mutants, using combinations of the conditions above, on the number of inclusions found will be studied. Direct interacting ability of proteins will be determined using a cell line and test tube models.
Research Design and Methods
1. To develop an animal model of ALS with OPTN:
Rationale: An animal model is necessary to further understand the pathogenesis of ALS associated with the OPTNE478G mutant, as has been done with other mutations such as SOD1G93A (15). A transgenic mouse model will be used instead of other models because it has been used before with OPTNE50K, the mutant associated with glaucoma (10), and the mouse homolog is more similar to the human OPTN gene than a fly or worm (27). A primate model will not be used, although 98% of the OPTN gene is consistent with the human gene (27), as typically a primate model is only used when a simpler model, such as a mouse, is not sufficient (8). The mutation is expected to be a gain of function mutation because OPTNE478G has been found in SOD1 and TDP-43 inclusions, indicating that the optineurin protein is over-expressed and thus presents itself in inclusions (19).
Design and Method
1. Developing a transgenic mouse and a knockout mouse and verifying the litter: A control mouse will be acquired that has no manipulation done to its DNA. Two transgenic mice will then be created using the CMV early enhancer/chicken beta-actin (CAG) promoter (10). The variants created will be the human OPTNwt and OPTNE478G, which correlates to ALS (19). The transgene will be created with the cloned promoter and human gene (3). Next, we will create a knockout mouse by deleting the coding sequence for optineurin (3). To then be sure that the procedures worked, Northern Blot analysis (3) and Western Blot analysis (37) will be used to check for the mRNA and protein respectively. Prediction: We expect to see no protein/mRNA in the analysis for the knockout mice, the normal protein/mRNA in the two control groups, and over expressed protein/mutant mRNA in the OPTNE478G transgenic mouse. If something other than this is seen, then the desired results of the transgenic/knockout mouse production were not achieved.
2. Studying the behavior and pathology of the mice to determine if they develop ALS: The mice will be tested once a week for weight and for footprint analysis regarding step length to monitor disease progression (17). Footprint analysis will be used instead of rotarod tests because it requires less time to train the mice. Two defining characteristics of ALS are inclusions and motor neuron death. Motor neuron death will be studied using motor unit number estimation (MUNE) (29; 32) to see the number of motor neurons present in the four groups of mice. As ubiquitin usually is also seen in inclusions (19), we will use ubiquitin immunohistochemistry to examine the brains of the mice and determine if inclusions form containing OPTN (34). Prediction: Over time, the OPTNE478G mice will perform much more poorly on the test, will weigh less, and will exhibit motor neuron death and inclusions, indicating disease progression, while the other mice will show no difference on the weight and behavior test and will not exhibit disease pathology. If the knockout mice show poor performance on behavioral tests and exhibit disease pathology, a loss-of-function mutation would be suggested.
3. Looking at NF-kB in the transgenic mice: We plan to look at NF-kB activity using a quantitative atomic force microscopy (AFM) technique, whose analysis provides the amount of NF-kB per µm. It also allows for an accurate model of quantifying NF-kB activity from a small sample such as a biopsy (unlike ELISA or EMSA) (20). Additionally, immunohistochemistry will be used to verify the above results using a NF-kB antibody to tag for NF-kB (36). Prediction: Because the wild-type OPTN is similar to NEMO and inhibits the TNFa activation of NF-kB (19; 38; 31), but OPTNE478G has been shown to disinhibit NF-kB (19), we expect to see the NF-kB activated in the mutant mice and inhibited in all other mice. If these results do not occur, then the OPTNE478G transgenic mouse is not a good model for ALS.
2. To evaluate differential regulation of motor neuron genes by Wild Type and mutant optineurin:
Rationale: It was recently discovered that SOD1G93A, a common mutation in ALS patients, increases the expression of motor neuron genes involved in the transcriptional process and activation of the NF-kB pathway (13). Hence, we believe that other mutations in ALS-related genes such as OPTNE478G could also affect motor neuron gene expression. We suspect that OPTNE478G and OPTNwt have a differential effect on motor neuron gene expression. Additionally, although optineurin’s promoter (31) and ubiquitin binding domain (38) are implicated in optineurin’s interaction with NF-kB, it is not clear whether optineurin and NF-kB interaction could also be indirect.
Design and Method
1. The effect of OPTNE478G on motor neuron genes expression: We will compare motor neuron gene expression between OPTNwt (control) and OPTNE478G by using a microarray assay (25) in conjunction with laser-capture microdissection(LCM), previously used to identify motor neuron-specific changes in gene . Approximately 60ng of RNA will be extracted from wild-type and OPTNE478G mice spinal cord and brain tissue by using the PicoPure RNAExtraction kit, from Applied Biosystems (13). The microarray assay will be performed using the Affymetrix GeneChip Mouse Genome 430A 2.0 Array from Affymetrix (13), which contains 14,000 mouse genes (1). We will confirm OPTNwt and OPTNE478G motor neuron gene expression by performing a Northern Blot analysis by using the random primer DNA labeling kit, from Roche Applied Science, and 32P-labeled dCTPto label the cDNA probes(18). These experiments will be coupled with a Western Blot that will allow us to observe the proteins that are being produced by the genes identified in the microarray assay (37; 25). Prediction: We expect to observe different hybridization of OPTNE478G and OPTNwt on the motor neuron genes involved in the transcriptional process and activation of the NF-kB pathway (13). If this is not seen, that would indicate that the mutation does not have a significant effect on motor neuron gene expression.
2. Characterization of optineurin’s interaction with NF-kB: To study the possibility of an indirect interaction between optineurin and NF-kB, we will create a deletion mutation on optineurin’s ubiquitin binding domain using a PCR based mutagenesis approach (25; 33; 24) In addition, we will also create a three nucleotide mutation on a putative NF-κB site in optineurin’s promoter (~1kb upstream of optineurin DNA sequence) by using PCR and the restriction enzymes HindIII and XhoI (31). Then, we will co-immunoprecipitatethe mutated optineurin and non-mutated form of optineurin (control) with an optineurin specific antibody (ab23666) from Abcam. We will verify co-precipitation of NF-kB with mutant and non-mutant optineurin by performing a pull-down assay (21). Lastly, we will verify the presence of NF-kB and its co-precipitation with optineurin by performing a Western Blot analysis (37; 31). Prediction: Because optineurin’s promoter and ubiquitin binding domain are involved in the interaction of optineurin with NF-kB, we expect to observe co-precipitation of optineurin and NF-kB only when using the non-mutated optineurin(38; 31). If we see optineurin and NF-kB binding when using the mutated form of optineurin then it is possible that cofactors might be involved in the binding between optineurin and NF-kB.
3. Understanding the relationship between optineurin proteins to FALS proteins, including SOD1 and TDP-43:
Rationale: Transgenic mice with OPTNE478G, SOD1A4V, and TDP-43A315T will be used to model ALS. The OPTNE478G transgenic mice will be used from the first aim because the aim will determine OPTNE478G ability to form a successful model organism with ALS. SOD1A4V and TDP-43 A315T transgenic mice have previously been used as effective ALS model organisms and both mutation types are most commonly found in ALS pathology (14; 35). These animals will help us to have the tools to better understand the roles between SOD1 A4V and TDP-43 A315T proteins in relation to OPTNE478G.
Design and Method
1. Determining the presence of OPTN in SOD1 and TDP-43 inclusions: We will be detecting OPTNE478G in SOD1A4V and TDP-43A315T transgenic mice using immunohistochemistry (19). The transgenic mice will be ordered from Animal Genomic Services (2). First the animals will be perfused transcardially (5; 19). Second, the spinal cord and brain tissues will be removed after overnight fixation in paraformaldehyde (5; 19). Then, SOD1 will be tagged using an N-terminus binding antibody; TDP-43 will be tagged using antibody TDP-43, where it will bind to exon 6 in the TARDP gene (4). Finally, the antibody OPTN-C will be used to tag for OPTN in the C-terminus of the protein (19). Prediction: Immunohistochemistry will reveal SOD1 inclusions and TDP-43 inclusions containing OPTNE478G proteins; or, if OPTN is not related to FALS then OPTN will not be present with SOD1 or TDP-43 inclusions.
2. Determining if the reverse is true: whether SOD1 and TDP-43 are found in OPTN inclusions: The OPTNE478G mice will be used from the first aim. Immunohistochemistry will again be used, but it will be used to detect for SOD1 and TDP-43 presence in OPTN inclusions (19). Similar procedures as above will be taken. Prediction: OPTN inclusions/protein clusters will compose of SOD1, TDP-43, or perhaps both types of protein inclusions. Again, if there is no relationship between OPTN and FALS then OPTN inclusions will not include SOD1 or TDP-43.
3. Determining the dependence of proteins on one another: The preparation will include mating SOD1A4V mice with OPTNE478G mice, TDP-43A315T mice with OPTNE478G mice, and SOD1A4V mice with TDP-43A315T mice. To determine whether mice were doubly mutated, immunohistochemistry will be used to determine the protein composition of selected progeny (30). The animal behavior and brain pathology of the progeny mice will be checked for ALS pathogenesis as was completed in the first aim. immunohistochemistry on these doubly mutated mice, similar to the procedure followed above. Prediction: Double mutations will result in a greater number of inclusions and interactions between proteins in comparison to the single mutated transgenic mice. Alternately, the double mutations would not increase the severity of the pathology and therefore the number of inclusions would be similar to single mutated animals.
4. Determining how well the proteins directly interact with one another: Two in vitro methods will be used to determine the extent of direct interaction: cell lines and test tubes. Desired protein products will be formed by inserting corresponding mutated gene vectors into E. coli—this will include SOD1A4V mice with OPTNE478G mice, TDP-43A315T and their respective wildtype forms (23). The cell lines will simply include the mutated genes in the E. coli vectors (22). The proteins which will be tested in test tubes will also include specific additions to the gene inserted in the E. coli vector, including histadine, GST, and FLAG, for SOD1, OPTN, and TDP-43 respectively (22). The cell lines will include the following set-ups: SOD1wt with OPTNE478G and OPTNwt with SOD1A4V. The same will be done with TDP-43A315T. Northern blotting and the addition of previously used antibodies will reveal the extent of protein interaction. The detection of interaction will be made in Northern blots with Nickel beads for the histadine bound SOD1 and anti-FLAG beads will capture or detect the interactions made with OPTN (23). Prediction: If the proteins in the cell lines interact with one another it will result in increased accumulation of protein product in the Northern blots, and the detection will be made with the appropriate antibodies. In the test tube, Northern blots of the proteins will form clumps on the gel, which will have appropriate beads to capture the given proteins. Large accumulations will indicate SOD1/OPTN or TDP-43/OPTN interactions. The proteins will not interact if such relationships do not exist.
References
1. Affymetrix. GeneChip® Mouse Genome Arrays Data Sheet. Retrieved 10/29,2010, from http://www.osa.sunysb.edu/udmf/ArraySheets/mogarrays_datasheet.pdf
2. Ags.med.yale.edu. (2010). Retrieved 10/01, 2010, from ags.med.Yale.edu.
3. Alberts, B., Bray, D., Hopkin, K., Johnson, A., Lewis, J., Raff, M., Roberts, K., & Walter, P. (2009). Essential Cell Biology. Madison Avenue, NY: Garland Science, Taylor & Francis Group.
4. Amyotrophic Lateral Sclerosis 10 with or without frontotemporal dementia with TDP43 inclusions; ALS10 (2010). Retrieved 10/01, 2010, from http://www.ncbi.nlm.nih.gov/omim/612069.
5. Azzouz, M., Ralph, G. S., Storkebaum, E., Walmsley, L. E., Mitrophanous, K. A., Kingsman, S. M., Carmeliet, P., & Mazarakis, N. D. (2004). VEGF delivery with retrogradely transported lentivector prolongs survival in a mouse ALS model. Nature, 429, 413-417. doi:10.1038/nature02544.
6. Braiser, A. R. (2006). The NF-kB Regulatory Network. Cardiovascular Toxicology, 6, 111-130.
7. Bruijn, L. I., Miller, T.M., and Cleveland, D. W. (2004). Unraveling the mechanisms involved in motor neuron degeneration in ALS. Annual Review Neuroscience, 27, 723-749.
8. Chan, A. WS. (2009). Transgenic primate research paves the path to a better animal model: are we a step closer to curing inherited human genetic disorders? Journal of Molecular Cell Biology 1, 13-14.
9. Chalasani, M., Balasubramanian, D., Swarup, G. (2008). Focus on molecules: optineurin. Experimental Eye Research, 87, 1-2.
10. Chi, Z.L., Akahori, M., Obazawa, M., Minami, M., Noda, T., Nakaya, N., Tomarev, S., Kawase, K., Yamamoto, T., Noda, S., Sasaoka, M., Shimazaki, A., Takada, Y., Iwata, T. (2010). Overexpression of optineurin E50K disrupts Rab8 interaction and leads to a progressive retinal degeneration in mice. Human Molecular Genetics, 19, 2606-2615.
11. Clement, A.M., Nguyen, M.D, Roberts, E.A., Garcia, M.L., Boillee, S., Rule, M., et al.(2003). Wild-Type Noneuronal Cells Extend Survival of SOD1 Mutant Motor Neurons in ALS Mice. Science, 302, 113- 116.
12. Donkervoort, S, & Siddique, T. (2001). Amyotrophic Lateral Sclerosis Overview. Gene reviews. Retrieved October 17, 2010, from http://www.ncbi.nlm.nih.gov/bookshelf/br.fcgi?book=gene∂=als-overview
13. Ferraiuolo, L., Heath, P.R., Holden, H., Kasher, P., Kirby, J., and Pamela J. Shaw. (2007). Microarray Analysis of the Cellular Pathways Involved in the Adaptation to and Progression of Motor Neuron Injury in the SOD1 G93A Mouse Model of Familial ALS. The Journal of Neuroscience, 27, 9201-9219. doi:10.1523/JNEUROSCI.1470-07.2007
14. Gitcho, M. A., Baloh, R. H., Chakraverty, S., Mayo, K., Norton, J. B., Levitch, D., Hatanpaa, K. J., White, C. L.,3rd, Bigio, E. H., Caselli, R., Baker, M., Al-Lozi, M. T., Morris, J. C., Pestronk, A., Rademakers, R., Goate, A. M., & Cairns, N. J. (2008). TDP-43 A315T mutation in familial motor neuron disease. Annals of Neurology, 63, 535-538. doi:10.1002/ana.21344
15. Israelson, A., Arbel, N., Da Cruz, S., LLieva, H., Yamanaka, K., Shoshan-Barmatz, V., & Cleveland, D.W.(2010). Misfolded Mutant SOD1 Directly Inhibits VDAC1 Conductance in a Mouse Model of inherited ALS. Cell, 67, 575-587.
16. Jiang, Y. M., et al. (2005). Gene expression profile of spinal motor neurons in sporadic amyotrophic lateral sclerosis. Ann. Neurol, 57, 236-251.
17. Knippenberg, S., Thau, N., Dengler, R., & Petri, S. (2010). Significance of behavioural tests in a transgenic mouse model of Amyotrophic Lateral Sclerosis (ALS). Behavioural Brain Research, 213(1), 82-87. doi:10.1016/j.bbr.2010.04.042.
18. De Marco, N., Buono, M., Troise, F., and Diez-Roux, G. (2006). Optineurin Increases Cell Survival and Translocates to the Nucleus in a Rab8-dependent Manner upon an Apoptotic Stimulus. The Journal of Biological Chemistry, 281, 16147–16156.
19. Maruyama, H., Morino, H., Ito, H., Izumi, Y., Kato, H., Watanube, Y., Kinoshita, Y., Kamada, M., Nodera, H., Suzuki, H., Komure, O., Matsuura, O., Kobatake, K., Morimoto, N., Abe, K., Suzuki, N., Aoki, M., Kawata, A., Hirai, T., Kato, T., Ogasawara, K., Hirano, A., Takumi, T., Kusaka, H., Hagiwara, K., Kaji, R., Kawakami, H. (2010). Mutations of optineurin in amyotrophic lateral sclerosis. Nature, 465, 223-227. doi: 10.1038/nature08971.
20. Menotta, M., Crinelli, R., Carloni, E., Bianchi, M., Giacomini, E., Valbusa, U., Magnani, M. (2010). Label-free quantification of activated NF-kappaB in biological samples by atomic force microscopy. Biosens Bioelectron 25, 2490-2496.
21. Nature Methods. (2004). Detection of protein-protein interactions using the GST fusion protein pull-down technique. Nature, 1, 275-276.
22. Oshima, S., Turer, E., Callahan, J., Chai, S., Advincula, R., Barrera, J., Shifrin, N., Lee, B., Yen, T., Malynn, B., & Ma, B. (2009). ABIN-1 is a Ubiquitin Sensor that Restricts Cell Death and Sustains Embryonic Development. Nature, 457, 906-909
23. Peters, G. A., Khoo, D., Mohr, I., & Sen, G. C. (2002). Inhibition of PACT-mediated activation of PKR by the herpes simplex virus type 1 Us11 protein. Journal of Virology, 76, 11054-11064.
24. Qi, D., and Scholthof, K.B. (2008). A one-step PCR-based method for rapid and efficient site-directed fragment deletion, insertion, and substitution mutagenesis. J. Virol. Methods, 149, 85-90.
25. Reece, R. (2004). Analysis of Genes and Genomes. England: John Wiley & Sons Ltd.
26. Rezaie, T., Child, A., Hitchings, R., Brice, G., Miller, M.C.P, Heon, E., Krupin, T., Ritch, R., Kruetzer, D., Crick, R.P., Safarazi, M. (2002). Adult-onset primary open angle glaucoma caused by mutations in optineurin. Science, 295, 1077-1079.
27. Rezaie, T., Waitzman, D.M., Seeman, J.L., Kaufman, P.l., Sarfarazi, M. (2005). Molecular cloning and expression profiling of optineurin in the rhesus monkey. Investigative Ophthalmology & Visual Science, 46, 2404-2410. doi: 10.1167/iovs.04/1243.
28. Rosen D.R., Siddique, T., Patterson, D., Figlewicz, D.A., Sapp, P., et. al. (1993). Mutations in Cu/Zn superoxide dismutase gene are associated with familial amyotrophic lateral sclerosis. Nature, 362, 59-62
29. Sartucci, F., Maritato, P., Moscato, G., Orlandi, G., Calabrese, R., Domenici, G., et al. (2007). Motor unit number estimation (MUNE) as a quantitative measure of disease progression and motor unit reorganization in amyotropic lateral sclerosis. International Journal of Neuroscience, 117(9), 1229-1236. doi:10.1080/00207450600936304.
30. Silhavy, T. J., & Gitai, Z. (2008). Sex to the rescue. Nature Methods, 5, 759-760. doi:10.1038/nmeth0908-759
31. Sudhakar, C., Nagabhushana, A., Jain, N., Swarup, G. (2009). NF-kB mediates tumor necrosis factor a-induced expression of optineurin, a negative regulator of NF-kB. PLoS One, 4, e5114. doi:10.1371/journal.pone.0005114
32. Van Dijk, J.P., Schelhaas, H.J., Van Schaik, I.N., Janssen, H.M., Stegeman, D.F., Zwarts, M.J. (2010). Monitoring disease progression using high-density motor unit number estimation in amyotrophic lateral sclerosis. Muscle Nerve, 42, 239-244.
33. Wang, W. and Malcolm, B.A. (1999). Two-stage PCR protocole allowing introduction of multiple mutations, deletions, and insertions using QuickChange site-directed mutagenesis. Biotechniques, 26, 680-682.
34. Wils, H., Kleinberger, G., Janssens, J., Pereson, S., Joris, G., Cuijt, I., et al. (2010). TDP-43 transgenic mice develop spastic paralysis and neuronal inclusions characteristic of ALS and frontotemporal lobar degeneration. Proceedings of the National Academy of Sciences of the United States of America, 107(8), 3858-3863. doi:10.1073/pnas.0912417107.
35. Williamson, T. L., Corson, L. B., Huang, L., Burlingame, A., Liu, J., Bruijn, L. I., & Cleveland, D. W. (2000). Toxicity of ALS-linked SOD1 mutants. Science (New York, N.Y.), 288, 399.
36. Xu, Z., Chen, S., Li, X., Luo, G., Li, L., Le, W. (2006). Neuroprotective effects of (-)-epigallocatechin-3-gallate in a transgenic mouse model of amyotrophic lateral sclerosis. Neurochemical Research 31, 1263-1269.
37. Ying H., Shen X., Park B., Yue, B.Y.J.T. (2010). Posttranslational Modifications, Localization, and Protein Interactions of Optineurin, the Product of a Glaucoma Gene. PLoS ONE, 5.
38. Zhu, G., Wu, C., Zhao, Y., Ashwell, J.D. (2007). Optineurin negatively regulates TNFa-induced NF-kB activation by competing with NEMO for ubiquitinated RIP. Current Biology, 17, 1438-1443. doi: 10.1016/j.cub.2007.07.041.